Creating Super Dentin: Using Flowable Composites as Luting Agents to Help Prevent Secondary Caries
Abstract:
As dental material science and technology rapidly evolve and enable dentists to render more efficient treatment, an old challenge persists—preventing the formation of secondary caries at the restorative margin. Research has indicated that the existence of an acid-base resistant zone (ABRZ), which forms between the adhesive layer and the tooth, may have a preventive effect. The ABRZ is also known as super dentin, and the thickness of this zone is material dependent. Functional monomers such as 10-methacryloyloxyethyl dihydrogen phosphate and materials such as flowable composites used as luting agents may play roles in formation of the ABRZ. This article also includes a case study to illustrate the author’s technique in helping to create the ABRZ.
All-ceramic restorations are increasingly being performed due to advances in dental material science and the popularity of computer-aided design/computer-aided manufacturing (CAD/CAM). In addition, dental laboratories have invested more in computerized technology used for fabricating restorations, and a growing number of clinicians are utilizing chairside CAD/CAM units. Despite these advances, the age-old problem of secondary caries occurring at the restorative margin remains. Recent studies have revealed the process for the degradation of the resin–dentin complex whereby the initial bond strength of dentin adhesives diminishes rapidly due to inherent enzymatic activity.1-7 Reports have addressed this dilemma by incorporating enzymatic inhibitors into the resin adhesive.8-12 Dental caries creates destructive cycles that begin with the production of an acid that demineralizes the dentin complex. The acid decreases pH levels and activates protein enzymes, which degrade the exposed collagen. This exacerbates the carious lesion.13 Therefore, the catalyst in dental caries progression is the production of an acidic environment that, in turn, activates enzymes capable of degrading the collagen matrix.14
Dental caries research has long been focused on preventing the progression of the initial caries and diminishing the risk for secondary caries. The major cause for failure of dental restorations is secondary caries.15 More attention has been given to changes in the formulation of dentin adhesives that have inhibitory effects on the destructive nature of dental caries and subsequent enzymatic degradation.16-18 Efforts have also been made in reducing technique sensitivity by diminishing the number of procedural steps. However, some reports suggest that along with achieving the benefits of timesaving steps by using a single-bottle self-etching adhesive, lower bond strengths have resulted.19,20 Increasing the resistance to acidic bacterial byproducts at the tooth–resin interface may effectively impede the progression of secondary caries.21
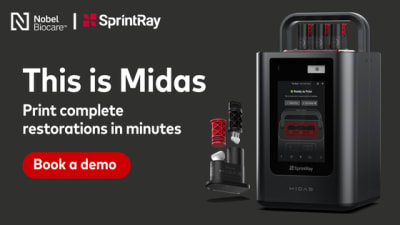
Why Buy Midas from Nobel Biocare
The marginal integrity of a dental restoration, whether direct or indirect, is subject to the efficiency of the bonding adhesive maintaining a marginal seal. Microgaps that form at the interface of the tooth and adhesive resin allow for the infusion of bacterial byproducts, leading to secondary caries.22 Manufacturers have modified dentin adhesive formulations to not only decrease the technique sensitivity of the procedure but also increase resistance to secondary caries formation with the creation of a zone that is acid-base resistant. This is referred to as “super dentin” and “super enamel.” The technology has led to changes in the insertion method used for anterior all-ceramic restorations. This article will discuss the tooth–resin adhesive-luting cement-restoration complex by first addressing tooth–resin adhesives and then examining luting cement restoration.
Super Dentin
The key to all adhesively bonded restorations is the formation of a hybrid layer.23 The hybrid layer is created after modification and/or removal of the smear layer, followed by demineralization of the dentin and penetration of adhesive monomers. Many studies have employed different techniques to characterize the hybrid morphology. For example, Nakabayashi23 applied hydrochloric acid-etching followed by sodium hypochlorite to demonstrate the acid resistance of the hybrid layer. Alternating between the application of an acid and a base material is known as an acid-base challenge. Nakajima et al24 used phosphoric acid followed by sodium hypochlorite in order to observe the hybrid layer, and Inokoshi et al25 utilized argon ion beam etching to visualize the hybrid layer. The scanning electron microscope (SEM) is another informative device employed to observe the ultrastructure of the hybrid layer.26,27
Using a SEM, Tsuchiya et al26 reported that the physical properties of the restorative and adhesive materials, the quality of the hybrid layer, and the release of fluoride had a preventive effect on the formation of secondary caries at the restorative margin. Their study found the presence of a thin layer adjacent to the hybrid layer that formed after an acid-base challenge; they referred to this layer as the acid-base resistant zone (ABRZ). In the acid-base challenge, each specimen was stored in a buffered demineralizing solution that contained a combination of calcium chloride, sodium phosphate, and acetic acid adjusted to a pH level of 4.5 for 20 minutes to create secondary caries. The specimens were then placed in a sodium chloride solution for 20 minutes and rinsed with water. The Tsuchiya study, as well as others,26-28 reported the formation of the ABRZ with the use of a self-etching primer adhesive system. They also observed the lack of the ABRZ when etch-and-rinse adhesive systems were used. Inoue et al29 found the existence of the ABRZ beneath the hybrid layer formed by a two-step self-etching adhesive system in both intact and caries-affected dentin.
Following the introduction of all-in-one adhesive systems, studies employing SEM and transmission electron microscopy (TEM) found the presence of the ABRZ beneath the hybrid layer, as well. In fact, a thicker ABRZ formed under the hybrid layer when the self-etching system was fluoride-releasing.30,31 The release of fluoride from conventional and resin-modified glass-ionomer cements has been reported to be anticariogenic.32,33 The incorporation of fluoride into resin adhesives has also been shown to have this effect.32,34 Nikaido et al have referred to the ABRZ as “super dentin” and the thickness of this zone is material dependent.17 Super dentin can be defined as a reinforced and modified dentin with superior resistance against caries activity.
The backbone of the self-etching dentin adhesive is the functional monomer, which has the role of improving bonding by increasing the wetting and demineralization of the dentin while chemically adhering to calcium.35 Yoshida et al36 found that a functional monomer did not completely demineralize the dentin but instead, left hydroxyapatite around the collagen, which served as a receptor for chemical bonding with the functional monomer. They also reported that the chemical bonding potential was different among functional monomers.
Super Enamel
The formation of an enamel ABRZ at the adhesive–enamel interface was confirmed by Li et al.15 They found this to only exist with adhesives that contained the functional monomer 10-methacryloxydecyl dihydrogen phosphate (MDP). Yoshida et al36 found that the MDP monomer interacted chemically with hydroxyapatite forming strong and stable ionic bonds with calcium. This reaction produced a MDP-calcium salt that had a lower solubility. The length and flexibility of the alkyl spacer chains have an effect on the polymerization reactivity of a functional monomer.37,38 The MDP monomer has a long linear alkyl chain and a phosphoric acid ester group that increases the polymerization efficacy.15 Nikaido et al39 reported the ABRZ in enamel was similar to that reported in dentin, although the enamel had a higher mineral content and a different matrix structure than dentin. In dentin, the ABRZ formed under the hybrid layer, while in enamel, the ARBZ formed along the adhesive–enamel interface. This enamel ABRZ was found to be more resistant than intact enamel and was referred to as “super enamel.”
Resin Adhesive and Cement Compatibility
Resin cement systems most commonly use etch-and-rinse single-bottle or all-in-one self-etching adhesive systems, which are acidic and hydrophilic.40 The acidity of the adhesive can present a compromise in the bond integrity. After placement and polymerization of the adhesive layer on the tooth substrate, an uncured oxygen-inhibited layer remains. Uncured acidic resin monomers are present in that layer. There is a competition between the acidic groups in the oxygen-inhibited layer of the adhesive resin that is bonded to the tooth and the peroxides that are in one of the dual-cure pastes for the tertiary amines that are present in the other dual-cure paste of resin cement.41 This competition results in a compromised copolymerization between the dual-cure resin cement and adhesive layer.42,43 The compromised effect is proportional to the time the cement cures; the longer the curing time, the more compromised the polymerization.40
To overcome this incompatibility issue, several manufacturers have introduced different types of chemical co-initiators or dual-cure activators (ternary redox catalyst).44 For instance, sodium salt of aryl sulphinic acid is commonly used45 as a co-initiator that reacts with the acidic resin monomer instigating polymerization by producing phenyl46 and benzenesulphonyl free radicals.47 Effective copolymerization was found to occur when these adhesives were bonded to water-free processed composites. When they were applied to hydrated dentin, a less-than-optimal copolymerization remained.48
The hydrophilic characteristics of these simplified adhesive systems can also compromise the bond integrity by allowing water to permeate through the adhesive after polymerization.48,49 If water is present at the adhesive resin cement interface, then the total bonded area is compromised and can result in adhesive cement failure.49 The presence of water can be traced to two sources: incomplete evaporation of water present in the adhesive itself (insufficient air-thinning of the adhesive prior to light polymerization)50; or the diffusion of water through the permeable adhesive from the underlying dentin.51 As a result, an intricate pattern of water-filled channels develops through the adhesive layer, referred to as water trees.52 As the water permeates through the cured adhesive layer via osmosis, osmotic blistering53 occurs. Some one-step self-etching dentin adhesives can undergo phase separation of the resin components, which can also lead to water on the surface.50
When a hydrophobic composite is applied to the cured adhesive surface, it acts to trap the water at the interface, forming water blisters,48 which act as mechanical stress raisers that disrupt the coupling between the adhesive and composite.54
Resin Cements
Every dental cement must meet basic standards prior to its approval for use in patient care. According to the International Organization of Standardization, resin cements must have a minimal flexural strength of 50 MPa, a water absorption rate less than 40 μg/mm3, and the same radiopacity as aluminum.55 From a clinical standpoint, resin cements must possess a lower viscosity than restorative composite resins, which allows for less resistance to fully seating a restoration. The viscosity, or rate of flow, depends largely on the amount and configuration of the filler particles. The filler material mechanically strengthens the resin cement by increasing the resistance to compressive and tensile forces.56 The sizes of the filler material in resin cements are divided into two groups: microfillers that are approximately 0.04 μ, and hybrid fillers that range from 0.7 μ to 1.7 μ.57 Christensen has stated that when comparing the two groups, research shows greater wear resistance for microfillers.58
Resin cements fall into two major categories: one that requires the application of a bonding agent prior to use and the other that is referred to as “all-in-one” resin cement and does not require a bonding agent. The latter is considered self-etching or self-adhesive.59 Although the self-etching cementing procedure consists of fewer steps, the bond strength has a lower potential.59,60
The polymerization of these resin cements is initiated either by light, chemical, or both means (dual-cure), and the selection should be based on each clinical situation. For example, in areas that have no access to light (eg, ceramometal crown), a chemical-cure resin cement should be employed. Dual-cure can be utilized in areas of limited light access, such as posterior all-ceramic restorations with deep interproximal extensions. Light-cure resin cement can be used in the anterior for all-ceramic crowns and veneers, provided the thickness of the restoration is less than 2 mm.61
Elastic Modulus of Luting Agents
The predominant factor in controlling the initial failure of ceramic is tensile stress, which is dependent on the differences in the elastic modulus of the ceramic, luting agent, and supporting material.62 A Hertzian cone indentation test shows how a ceramic reacts when a load (cone indentation) is applied to the surface. If the elastic modulus of leucite-reinforced ceramic is approximately 70 GPa and the supporting material—the dentin—is 16 GPa, then the elastic modulus of the luting agent should be as close to dentin as possible to minimize the mismatch. Radial (conical) fractures that result in bulk-ceramic failures develop more readily when the supporting substrate has a lower elastic modulus.
Habekost et al63 studied how the elastic modulus of three luting agents affected the fracture resistance of teeth restored with ceramic inlays. Considering the fact that the luting agents also differed in bonding strength, the researchers found improved fracture resistance for the ceramic-restored teeth that had the higher elastic modulus cement. Addison et al64 reported that resin cements have a strengthening effect on all-ceramic restorations. They found that the increased strength was proportional to the elastic modulus of the resin cement, which was dependent on the amount of filler loading. The influence of the resin coating on the biflexural strength of the ceramic increased as the elastic modulus of different resin cements increased. Öztürk et al65 showed that filler loading had the highest effect on elastic modulus.
Flowable Composites Used as Luting Agents
In general, flowable composites have a decreased filler concentration compared with restorative composites, which allows for an increase in the surrounding resin matrix. Most flowable composites are filled between 41% and 53% by volume, which is contingent on the type and shape of the filler material. The amount of filler material can also be expressed by weight, which is usually higher (ie, 41% to 53% by volume would equal 56% to 70% by weight).66 Weight is more commonly used by manufacturers because of the higher number. The increase in the resin content thereby increases the polymerization shrinkage upon curing.67 If the amount of filler content is increased, then the flow is minimized, which would decrease the polymerization shrinkage but increase the difficulty in fully seating a restoration when used as a luting cement. An ideal luting cement would have low polymerization shrinkage, high filler content for strength, and high flowability, allowing a restoration to be easily placed. The material should also have low solubility, as well as high compressive and tensile strengths. The proportional limit would be high and have excellent adhesion to the ceramic bonding surface and the adhesive–tooth interface.
Shrinkage of composite resins occurs when the material polymerizes. The amount of shrinkage is proportional to the filler type, shape, and volume of that material.68-71 As polymerization progresses, bulk reduction occurs as molecules come closer together, forming a polymer network.71-74 When a composite resin has a greater filler volume, molecules have less space to compact, thereby decreasing polymerization shrinkage.75 The elastic volume, volumetric shrinkage, and development of polymerization contraction stress are all dependent on the volume of filler particles.76 Masouras77 stated that in addition to the amount, size, and type of filler material, the coupling to the resin matrix to the filler particles played an important role in the material’s performance. Geometrically, a higher volume of filler particles of various sizes that are spherical can be incorporated into the resin matrix.78,79 Stresses tend to develop at the sharp edges of filler particles, which, in turn, decrease the fracture strength. The spherical filler particles tend to improve fracture strength.79
Hooke’s Law states that stress is equal to the volumetric shrinkage multiplied by the elastic modulus and that the greater the polymerization shrinkage and the higher the elastic modulus of a composite resin, the larger the contraction stress. Manifestations of stress (ie, shrinkage) can be observed in composite resin material as internal crack formation,80 flexure or deformation of the surrounding tooth structure,81-83 and/or the development of a gap between the tooth and the composite material after adhesive failure occurs.84
Because the filler content has a dramatic effect on polymerization shrinkage, the use of a flowable composite as a luting agent may seem less desirable. Munskgaard et al75 found a direct correlation between polymerization contraction stresses of resin-based composites to filler volume. The higher the filler volume, the lower the contraction stress, which, in turn, resulted in better wear resistance and marginal integrity. If the filler content of a flowable composite was in the range of a sculptable restorative composite (70% to 80%) and shared the same physical properties, it may be a desirable substitute for resin cement.
Flowable composites have been suggested for use as an alternative in cementing ceramic inlays and veneers.85 Barceleiro et al61 found similar results when bonding feldspathic porcelain to bovine enamel using a dual-cure resin cement and a light-cure flowable composite. Their study suggested the use of flowable composites as a suitable alternative luting agent when bonding porcelain laminate veneers that were less than 2 mm in thickness. Prieto et al86 studied nanoleakage patterns of leucite-reinforced ceramic bonded to enamel using different resin systems and flowable composites. They concluded that the use of a two-step etch-and-rinse adhesive with a flowable composite as a luting agent created an adequate seal at the bond interface at the enamel.
Not all flowable composites are the same. There is a wide variety of flowable composites with a range of physical properties that include flow, flexural strength, modulus of elasticity, and radiopacity,86 as well as filler content. The differences in radiopacity of flowable composites are equivalent to the range in opacity between enamel and dentin. Flexural strengths have been reported to be as low as 66 MPa87 and as high as 145 MPa (manufacturers’ data). Elastic moduli span between 2.8 GPa87 and 10.5 GPa (manufacturers’ data).
Table 1 compares the filler content, compressive strength, flexural strength, elastic modulus, and water sorption value of two notable flowable composites that have filler contents higher than 80% by weight (62% by volume) and four veneer cements.88 These resins have differences and similarities but most notable is the elastic modulus and the water sorption value. The significance of the physical properties listed in Table 1 is fairly understandable; however, the effect of water absorption by resin composites may not be observed for some period and yet has a critical role in the success of the restoration.89,90
Water Sorption of Resin Composite Materials
Water sorption and solubility properties of resin composite cements play an important role in their clinical performances in terms of dimensional changes and structural integrity.91 Over time, water sorption causes a degradation of the flexural strength of resin composites,92 as well as an increase in marginal leakage,93,94 leading to secondary caries.95 In a moist environment, the resin matrix can absorb water and swell, causing a decrease in the elastic modulus.90 The absorbed water acts as a resin plasticizer and diminishes the support to the overlying ceramic restoration, leading to an increase in fractures under biting forces.96 The greater the amount of resin matrix, the higher the volume of water absorption.91 The absorption of water by the resin matrix can produce hydrolytic degradation of the filler–resin interface.97 Other factors in addition to filler concentration influence water sorption and solubility. These include the nature of the filler particles, the mean particle size, and the bond between the filler and matrix or the coupling agents.89
Therefore, all-ceramic anterior restorations that are less than 2 mm in thickness can be inserted with a low-viscosity light-cure-only, highly filled, flowable composite resin that has a higher modulus of elasticity and a low water sorption value. Coupling a light-cure-only flowable composite with a light-cure-only adhesive that has the capability of creating an ABRZ that decreases secondary caries potential can be a viable cementation protocol.
Case Study
A 40-year-old woman presented with a history of laminate veneers having been placed on her two maxillary central incisors. Her complaint was the discoloration of these older restorations, the gingival recession that had resulted in displaying the root surface, and the disparity in length between the centrals, lateral incisors, and canines (Figure 1 through Figure 3). She wanted more uniformity in color and length of the six anterior teeth. A mock-up was created on centric-related mounted models in the laboratory. A diode laser was used to alter the gingival margin contour of the right central incisor due to the irregular pattern of recession (Figure 4). Using a vacuum-form stent, the mock-up was bonded into place (total-etch on the entire facial surface) on the six anterior teeth after removal of the previous restorations. The shade of the mock-up composite resin (Prodigy™, Kerr Dental, www.kerrdental.com) was selected as a preview of the final shade of the ceramics (Figure 5 through Figure 7). Although the proposed treatment plan (mock-up) involved the patient having to make an additional appointment, she was able to “test-drive” the planned restorative scheme prior to preparations and confirm the esthetics, phonetics, and function.98 The patient wore the mock-up for 1 week and then approved the prosthetic change. A new alginate impression was made to preserve the prosthetic changes and would be used in the laboratory for duplicating purposes using the wax-injection method.99-101
With the mockup in place, round diamond burs of known dimension were used to place depth cuts on the facial and incisal edges of the six anterior teeth using the shaft of the bur against the facial contour of the tooth to control the depth. A round-ended diamond-tapered bur was used to remove mock-up material and/or tooth structure between the depth cuts. This method accurately removes the minimal amount of mock-up material or tooth structure that is required for the manufacturer’s (Ivoclar Vivadent, www.ivoclarvivadent.us) ceramic thickness requirement.102,103 Any mock-up material that remained after the initial reduction was removed before the preparations were finalized. The lateral incisors and canines were minimally prepped because the mock-up material accounted for most of the final restoration’s volume (Figure 8). The teeth were impressed and temporalized using the same material used for the mock-up (Prodigy).
In the laboratory, the wax injection technique was used to create the laminate veneer restorations in wax. A silicone mold made from the mock-up model worn by the patient was placed on a solid working model. An injection hole was placed at the incisal edge of both canines. Melted wax was injected under pressure into one of the holes in the silicone mold continuously until it extruded from the other hole. The wax cooled, and then the silicone mold was removed, leaving a wax replica of the mock-up. The wax laminate veneers were separated individually and placed on another multiple-die working model to finalize the margins. The wax-ups were then invested and pressed using IPS e.max® Press LT (Ivoclar Vivadent). After devesting the restorations, the incisal one-third of the laminate restorations was cut back and layered in a series of steps with a combination of incisal porcelains. The restorations were finished, using a series of diamond-impregnated wheels and then polished with Zircon-Brite (Dental Ventures of America, Inc., www.dentalventures.com). This polishing material created a natural appearance without requiring the use of a glazing porcelain layer. The restorations were then steam-cleaned, but bonding surfaces were not treated at this time.
At the insertion appointment, the provisional restorations were removed. The surfaces of the prepared teeth were cleaned using an air-abrasion unit (Figure 9). The restorations were tried in, using water instead of a try-in paste. The patient viewed the restorations in place and approved the result. The restorations were water-rinsed and dried. A 9% hydrofluoric acid was applied to the intaglio surface for 20 seconds, water-rinsed, and dried. A ceramic primer containing the MDP monomer (Clearfil™ Ceramic Primer, Kuraray, www.kuraraydental.com) was then applied to the bonding surface and air-dried. A resin adhesive (Clearfil™ S3 Bond Plus, Kuraray), which also contained the MDP monomer, was applied to the bonding surface and air-thinned to accelerate evaporation of water and solvents (Figure 10). Clearfil™ Majesty™ Flow (E2) (Kuraray) was then applied to the bonding surface of the restoration (Figure 11), which was then placed in a Calset Veneer warming tray (AdDent Inc., www.addent.com) and covered (Figure 12). Increasing the temperature of the flowable composite eases the placement of the restoration and increases the degree of conversion of the resin. 104-109
The prepared teeth were isolated and dried. The overhead unit light was turned off. A self-etching fluoride-releasing one-step adhesive (Clearfil S3 Bond Plus) was applied to each prepared surface for 10 seconds without agitation (Figure 13). This resin adhesive has been shown to produce an ABRZ layer. The adhesive was then air-thinned (Figure 14) and light-cured for 20 seconds per tooth. As previously mentioned, phase separation can occur when using some one-step self-etching dentin adhesives. The manufacturer describes this all-in-one resin adhesive as having a homogenous mixture of hydrophilic and hydrophobic components using molecular dispersion that prevents phase separation and water formation on the adhesive surface.
The preloaded warmed veneers were placed starting with the central incisors followed by the lateral and then the canines. A variation of the light polymerization method referred to as the “Tack and Wave” technique110 was employed. Both central incisor veneers were secured in place using simultaneous light pressure in an incisal direction with the index fingers and labial pressure with the thumbs. An LED curing light with a 2-mm light guide was placed at the center of each veneer and activated for 1 second on the facial side. The same procedure of securing and tacking down the restoration was repeated for the lateral incisors followed by the canines. The light guide tip was changed to a larger diameter. Holding the light tip 1 inch from the labial surface, further light activation was completed using a waving motion for an additional 4 seconds on the labial side and then on the lingual side. The excess flowable composite was then removed with a 12-b surgical blade mounted in a round handle instrument. Dental floss was passed through each interproximal contact. A clear water-soluble gel was used to cover all margins to prevent the formation of an oxygen-inhibited layer during the final light-curing step. Using two curing lights, one placed on the facial and the other placed on the lingual, the veneers were light-cured for 60 seconds divided into 20-second increments, preventing overheating of the tooth.
The margins were checked and finished using ultra-fine diamond burs. Any remaining resin on the veneer surface was removed with the 12-b surgical blade, and then the restorations were polished with a soft bristle brush and Zircon-Brite polishing paste (Figure 15). The patient was given postoperative instructions and was instructed to return in 2 weeks for follow-up and photographs (Figure 16 and Figure 17).
No published randomized clinical trials regarding the author’s technique involving creation of an ABRZ combined with flowable composite as a luting agent have been published, and the hypothesis is still theoretical. Although promising, more research is indicated.
Conclusion
Many dental researchers and manufacturers share a goal of wanting to simplify technology to produce better outcomes. Secondary caries forming at the marginal edge and ceramic fractures are two factors that affect the longevity of indirect dental restorations, making these the most common reasons for the need to replace restorations. The selection of bonding adhesives and the techniques employed are critical to the integrity of the tooth-resin-restorative complex. Providing an environment that can resist more readily the damaging effects of bacterial byproducts at restorative margin is an example of advancements in dental material science. Although more research is needed, the results are promising. The technologic progress made in resin adhesives, techniques, and luting materials is increasing the lifespans of these restorations.
ACKNOWLEDGMENTS
The author would like to thank Ruth Egli, RDH, for her editorial contribution. All laboratory work was done by the author.
About the Author
Gregg A. Helvey, DDS
Adjunct Associate Professor
Virginia Commonwealth University School of Dentistry
Richmond, Virginia
Private Practice
Middelburg, Virginia