Resin Composite Restorations: Innovations and Technology Continue to Drive Success
Contemporary dentistry cannot be performed without resin-based restorative materials, light-curing units, and sectional matrix band systems.
The current tendency towards "minimally invasive dentistry," driven in part by a growing demand for overall esthetics, has established resin composites as the preferred material for anterior and posterior tooth restorations. Contemporary dentistry cannot be performed without resin-based restorative materials, light-curing units, and sectional matrix band systems.
Developments in Resin-Based Composites
Resin-based composites (RBCs) are widely used for a range of applications, such as restorations, sealants, core buildups, inlays, onlays, crowns, posts, cements, as well as in orthodontics and endodontics.1 These materials involve four main components: an organic matrix, an initiator system, a coupling agent, and inorganic filler particles.2 While resin monomers have largely remained unchanged, significant advancements have been made in the type, size, and distribution of the inorganic fillers. Over the years, the development of nanotechnology and the incorporation of nanofillers greatly enhanced the quality of RBCs, resulting in better mechanical properties, better handling of the material, and improved esthetics as smaller size particles reduce surface roughness, which improves color stability and glossiness and reduces bacterial adhesion.3 Today, these types of materials represent the state of the art in terms of filler formulation.
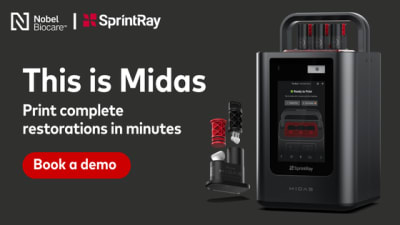
Why Buy Midas from Nobel Biocare
Achieving clinical success is highly dependent on restorative materials that blend flawlessly with the surrounding tooth structure, creating a "chameleon effect." Traditionally, multilayering techniques are used to match tooth shades, but these methods require high skill levels and are time-consuming. Single-shade composites have been developed to simplify the process. These new compositions aim to accomplish this effect by increasing translucency, facilitating tooth color matching and optical blending with a single "universal" shade (eg, Omnichroma®, Tokuyama, tokuyama-us.com; SimpliShade™, Kerr, kerrdental.com; Admira Fusion X-tra, VOCO, vocoamerica.com).4
Nevertheless, concerns about RBCs include polymerization shrinkage, gap formation, bacterial leakage, secondary caries, crack propagation, and fracture.1 Current efforts are mainly focused on optimizing the organic matrix to reduce both polymerization shrinkage and post-polymerization stress. Bulk-fill composites represent a low shrinkage stress material due to a higher concentration of photoinitiators, reduced filler loading, and increased translucency. Short fiber-reinforced composites are aimed at increasing stress absorption and fracture toughness and lowering polymerization shrinkage stress and crack propagation with the incorporation of randomly oriented glass fibers and fillers into the polymer matrix (eg, everX Posterior, GC Corporation, gc.dental) for use as a bulk substitute for dentin by mimicking its stress-absorbing properties.5,6
In addition to direct filling materials, resin-based CAD/CAM prefabricated blocks (chairside and laboratory) are also available for indirect partial- and full-coverage restorations. These materials consist of a pre-sintered resin-infiltrated system with a high filler volume, resulting in improved mechanical properties. Compared to ceramics, they experience less crack formation during manufacturing, better marginal integrity after milling, and are easier to adjust and repair (eg, Cerasmart®, GC Corporation; Lava™ Ultimate, 3M Oral Care, 3m.com).2
Currently, a limited number of composite materials are available for 3D printing indirect restorations using digital light processing or stereolithographic technology (additive manufacture). These RBCs are still being developed as mechanical properties such as bond strength, microhardness, and wear resistance are deeply influenced by the resin's composition, production parameters, and post-production processes, including cleaning and curing. There is insufficient data on their clinical performance to support any evidence-based recommendations for their use in clinical settings at this time.7,8
As for future developments in RBCs, current investigations are focused on developing polymeric materials that exhibit low polymerization stress, high toughness, resistance to hydrolytic and enzymatic degradation, the ability to eliminate or inhibit bacterial biofilms, and self-healing properties, with the aim to enhance the clinical longevity of adhesive dental restorations. Nanotechnology has enabled the creation of dental materials with antibacterial properties through contact-active technology. Hydroxyapatite nanorods serve as effective reinforcement material for RBCs, improving enamel biomineralization, and are also known for their antimicrobial properties.9 Zeolites, when loaded with calcium, can provide anticaries activity, while silver-based coatings are also popular due to their antibacterial effects and biocompatibility.1,4 Graphene serves as a reinforcing filler and has been shown to have a broad antibacterial spectrum while maintaining bulk mechanical properties. It is also used for dentin hypersensitivity. Because of its grayish color, white-shade graphene has been developed with similar properties.2
The next generation of RBCs is envisioned to move from an inert cavity filling towards a more dynamic interaction with the oral environment. These materials could incorporate uniquely coded instructions within their chemical structures, enabling them to perform specific functions as needed.
Choosing the Right Curing-Light Unit
One critical step following the placement of RBCs is the polymerization phase, which is significantly influenced by the type of curing light and the curing mode used.
Light-cured RBCs include a photoinitiator (usually a camphorquinone) and an aliphatic amine activator (dimethylaminoethyl methacrylate). A major well-known disadvantage of light-cured RBCs versus self-cured composites is polymerization shrinkage and the associated stress generation that may lead to clinical restoration failure.10 If an RBC is not adequately cured, it will fail to achieve its optimal strength and proper bonding to the tooth structures. This can lead to microleakage, postoperative sensitivity, secondary caries, and potential fracture. Other factors, such as proper handling of the light-curing unit, internal composition of RBCs, and environmental conditions, can further impact polymerization.
Different types of curing lights and curing modes have been shown to affect the degree of polymerization and related shrinkage of RBCs. Currently, four types of blue light sources are being used to activate the photoinitiators found in dental resins: quartz-tungsten-halogen (QTH), plasma arc (PAC), argon ion laser, and light-emitting diode (LED). These sources produce photons of light in different ways and deliver different emission spectrums and wavelengths of light.11
QTH lights use a quartz bulb with a tungsten filament in halogen gas, emitting a spectrum of visible light ranging from 200 nm to 550 nm by a filter. In contrast, LED lights emit a narrower wavelength tailored for activating photoinitiators.12 LED technology has become the standard for dental curing lights. Most dental LED units are equipped with an LED chip that emits a blue light at around the 465-nm wavelength, and most recent units include additional chips that emit a violet light at wavelengths of approximately 405 nm (polywave curing lights).
When polymerizing RBCs, the efficiency of the light-cure unit is influenced by multiple factors, such as the photoinitiator type, bulb intensity, wavelength of the emitted light, exposure time, distance to the composite surface, inclination angle of the light tip, as well as the composite's shade and type. A recent innovation in RBC technology is fast-curing composites, which can be polymerized in as little as 3 seconds using a 3,000 mW/cm² curing light. This rapid curing, enabled by a different photoinitiator (Lucirin-TPO), reduces operator inaccuracy and achieves high conversion rates with minimal exposure time. The use of high-power intensity for a short period of time has been shown to decrease cytotoxicity, emphasizing the beneficial effect of light-curing methods on RBC biocompatibility.4,13
Advancements in Matrix Band Systems
Recreating tight proximal natural and anatomical tooth contour and function is a significant challenge most clinicians encounter when placing a Class II and III RBC restoration. This can be achieved using a matrix band system. The purpose of a matrix band is to create a mold for the missing tooth wall and provide restraint for the filling material. Deficiencies in the interproximal contact area can significantly affect surrounding tissues, generate food impaction and masticatory discomfort, and lead to recurring caries and periodontal complications.14,15
Traditionally, matrix bands were created from thin, flexible, flat pieces of metal and placed circumferentially around the tooth.16 Today, advancements in modern materials and techniques give clinicians a variety of matrix choices to recreate an anatomically correct contour with a tight contact area, sufficient marginal adaptation, and precise marginal ridge placement. Most sectional matrices are made of steel or acetate. Clinical and in vitro studies show no statistical difference in marginal seal quality between metal and acetate matrices.17 Several techniques and instruments have also been explored to establish optimal proximal contacts, including pre-wedging, condensable composite materials, and proximal box-forming tools. Nevertheless, none of these methods have demonstrated the efficacy of consistently reproducing a secure contact area.15
Currently, sectional matrix systems combined with separation rings offer the best proximal contact tightness for Class II and III RBC restorations.14,15,18,19 Circumferential band matrices are less efficient in forming correct contact areas due to their non-anatomical nature when compared to sectional matrices with multiple convexities. The emergence profile created with circumferential band matrices is flat and more vertical, leading to the relocation of the contact area in a higher position near the occlusal surface and displacement of the marginal ridge crest toward the adjacent proximal wall, creating a higher risk for fracture.
Conclusion
Selecting the appropriate resin-based composite, a suitable light-cure unit, and sectional matrix band and ring is essential for a successful restoration, as these materials and systems have different formulations and characteristics, each with its own unique strengths and weaknesses.
About the Authors
Macarena Rivera, DMD, MSc
Assistant Professor, Department of Prosthodontics, University of Chile, Santiago, Chile; Adjunct Professor, Department of Preventive and Restorative Sciences, University of Pennsylvania School of Dental Medicine, Philadelphia, Pennsylvania; Private Practice, Santiago, Chile
Nikolaos Stefanos Kampanas, DDS
National and Kapodistrian University of Athens, School of Dentistry, Athens, Greece; Private Practice, Athens, Greece