Effect of Surface Treatment on 3D-Printed Ceramic-Filled Hybrid Material With Self-Adhesive Luting Cement
Self-adhesive luting used to bond 3D-printed resins improves the efficiency and accuracy of these materials.
ABSTRACT
Objective: The aim of this study was to compare the bond strength of 3D-printed ceramic-filled resin in relation to surface treatment. Materials and Methods:3D-printed ceramic-filled hybrid resin block specimens were divided into two groups, G1 and G2, depending on whether air-abrasion was used or not, and bonded to using a self-adhesive luting resin according to the manufacturer's instructions and thermal-cycled for 5,000 cycles at 5ºC to 55ºC. After thermal-cycling, the specimens were subjected to the shear bond strength (SBS) test until fracture occurred. Data were analyzed using student t-test for independent samples (SBS) and chi-square test to analyze failure type (P < .05). Results:In the SBS test, no statistically significant differences were observed (P = .062) with regard to a higher adhesion strength in G1 (air-abraded surface) compared to G2 (non-air-abraded surface). Chi-square test showed statistically significant differences (P < .001) related to failure type. Conclusions:The shear bond strength of the ceramic-filled hybrid material seems to be adequate for clinical applications, while abrasion by airborne particles with 50 μm of aluminum oxide improves the bond strength. Clinical Significance:Self-adhesive luting used to bond 3D-printed resins improves the efficiency and accuracy of these materials. These cements reduce the need for preconditioning, which simplifies cementation and reduces clinical time.
In recent years technological advances in dentistry have simplified manufacturing techniques for definitive restorations. Adhesive techniques and computer-aided design/computer-aided manufacturing (CAD/CAM) are currently used to help facilitate minimally invasive restorative dentistry.1 More recently, the use of additive systems for clinical applications has grown exponentially, especially with the development of new photopolymerization liquid polymer-based resins.2
Since the early 1990s, 3D printing techniques have been gradually implemented in dentistry with several materials developed for intraoral application. The first systems introduced were able to print metals, while the usage of polymers has increased of late.3-5 Clinically, these materials must not only provide the required precision but also possess the necessary biological and physical properties.6
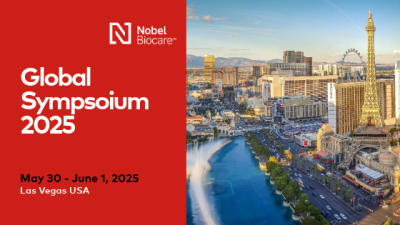
Nobel Biocare Global Symposium 2025
Additive manufacturing, or 3D printing technique, has been developed as a fast manufacturing tool that competes with conventional laboratory-processed and hybrid restorations that are milled or produced via subtractive manufacturing.4-8 When comparing additive manufacturing to subtractive manufacturing, the amount of material required for a definitive restoration using the former method is much less, as is the time and cost of fabrication.1,3,8,9
According to the international standard ISO/ASTM 52900:2023, additive systems are divided into seven categories, and in dentistry the most used system is vat photopolymerization.5,10 However, it is important to have an appropriate post-processing strategy to avoid compromising the mechanical properties of the material. Manufacturers may have different post-processing strategies for cleaning objects and removing monomer residues from their surfaces. It is generally recommended to wash the object in an ultrasonically activated ethanol bath to remove the monomer layer. Nevertheless, some manufacturers suggest using centrifugal force to remove the monomer. Regarding post-curing, various polymerization units are available in the market.11,12
Flow consistency and structural stability during print and storage are two of the most important characteristics for 3D printing materials. Similar to flowable dental composites, printable dental materials for permanent restorations should have less organic filler than composites that are offered in blocks or discs. Decreased filler content reduces the hardness of the material and may result in a significantly lower elastic modulus value than hard tissues.1,13
3D-printed resin-based temporary materials are appropriate for long-term use and have obtained approval under medical device regulations for use as materials for fixed dental prostheses.12,14 However, there is a scarcity of literature on a novel ceramic-filled hybrid material (VarseoSmile Crown plus, Bego, bego.com) that offers improved properties that allow it to be used as a definitive restoration over conventional 3D-printed resins, which have been primarily used for temporary restorations. Nonetheless, the material's mechanical properties and clinical performance have not yet been sufficiently validated.
A key factor for the success of a restoration is the surface treatment of the tooth by chemical or mechanical methods. In order to increase the surface energy some surface treatments such as silicon carbide paper grinding, air abrasion, diamond bur cutting/grinding, or the use of hydrofluoric acid may be employed.8,14,15 Some studies have shown good results using air-abrasion with aluminum oxide at 1 to 2 bar pressure.16-18 The mechanical and chemical properties of dental materials determine their cementation mode. In general, dental materials can be divided into two groups based on their cementation: passive materials, which are retained by mechanical friction (conventional cementation), such as zinc phosphate cements or glass ionomers, and active materials, which are those whose chemical composition interacts with the surface of the material to allow a functional bond between tooth and restoration, such as resin luting cements for bonding techniques.19-22
Resin luting cements are frequently used instead of traditional cements due to their ability to create a stable bond between restorations and abutments, resulting in improved mechanical properties. Resin luting cements can be classified based on their chemical curing reaction or surface treatment required before application. Chemical curing cements are divided into light-activated, self-cured, and dual-activated resin cements. Depending on the surface treatment prior to their application, options are conventional resin cements, which require enamel and dentin pretreatment, self-adhesive resin cements, and self-adhesive cements with a dental primer. Conventional resin cements require several steps before cementation, resulting in a technically sensitive procedure. In contrast, self-adhesive resin cements do not require pretreatment of the tooth structure because they contain acidic functional monomers that demineralize the tooth surface, allowing resin penetration.22-24
For restorative materials, one of the most commonly used mechanical treatments to increase the bond strength surface and create better mechanical interlocking prior to cementation is the use of air-abrasion, which uses powders with varying sized particles and pressures as described in the literature.12,15,22,25,26 As some authors have highlighted, few studies have evaluated the effect of air-abrasion on the surface of 3D-printed materials. Lankes et al evaluated the influences of air-abrasion and the cleaning system used.12
Because of the scarcity of literature on this topic, this study aimed to compare the bond strength of self-adhesive luting cement on ceramic-filled hybrid material used in 3D printing techniques, with and without air-abrasion surface treatment. The following hypothesis was investigated: there is an improvement in the shear bond strength (SBS) of the air-abraded blocks.
Materials and Methods
A sample size calculator was used to determine the ideal sample size.27 The sample size was established on 16 specimens in each of two groups, assuming an alpha risk of 0.5, a beta risk of 0.2, and a risk of dropout of 0.6.
Thirty-two 18 mm x 14 mm x 12 mm 3D-printed ceramic-filled resin blocks (VarseoSmile Crown plus) were printed with a digital light processing (DLP) printer (Varseo XS, Bego), with the parameters established by the manufacturer. After the specimens were removed from the build platform, they were ultrasonically cleaned in 91% isopropanol for 5 minutes. The post-cure process was performed in the curing device system (Otoflash® G171, NK Optik, nkoptik.com); in this case the power input was 250 watts and 10 flashes per second in a cycle of 1,500 flashes per each side. Blocks were divided into two groups: G1, air-abraded surface; and G2, non-air-abraded surface. Two specimens for each resin cement were attached to each block (n = 16) (Table 1 and Table 2). The bonding surface of each specimen was cleaned with alcohol before the bonding process.
Self-Adhesive System - Specimens' Preparation
According to Table 2, the group with surface treatment was air-abraded with 50-μm aluminum oxide particles for 20 seconds at 2 bar pressure, at a distance of about 5 mm from the specimen, and then dried for 10 seconds. The non-air-abrasion group was cleaned, dried for 10 seconds, and resin-cement specimens were bonded.
Resin Cement Specimens
The luting agent was directly applied in one 3-mm layer into a special mold and light-cured with a LED light-curing unit (Demi™, Kerr, kerrdental.com) for 40 seconds from the top and bottom to fabricate the specimens with an intensity of 1,200 mW/cm2. Resin cement was mixed and applied according to the manufacturer's recommendations. Excess resin was removed using foam pellets.
Storage and Aging of Specimens
After fabrication, the specimens were stored in 100% humidity at 37ºC ± 1ºC for 24 hours. Before submitting the specimens to the SBS test, they were aged in a thermocycling machine for 5,000 cycles at 5ºC to 55ºC with a dwell time of 30 seconds and transfer time of 10 seconds.
Shear Bond Strength Protocol
Specimens were subjected to force using a universal testing machine (Z005, Zwick/Roell, zwickroell.com) at a crosshead speed of 0.5 mm/min; the force was applied in the interface between the 3D-printed resin and the cement, until the fracture of the specimen. The SBS for each specimen was calculated by dividing the maximum load in newtons (N) by the surface area (4.52 mm2) to obtain the strength in MPa.
Evaluation of the Interface
The fracture surface of each specimen was examined under a stereomicroscope (SteREO Discovery.V8, Zeiss, zeiss.com) at 120X magnification to evaluate the failure mode, which was categorized as adhesive, cohesive, or mixed.
Statistical Analysis
The statistical analysis was carried out using the Jamovi statistical program (version 2.2.5) (Jamovi, jamovi.org). The Shapiro-Wilk test was performed to establish whether the sample distribution was normal for both studied variables (SBS and failure type). The student t-test for independent samples was conducted to analyze the relation of surface treatment in SBS data. A chi-square test was used to establish the significance level in failure type. A confidence level of 95% was used in all tests, and a significant difference was accepted at P < .05.
Results
After performing the student t-test for independent samples in the SBS test, no statistically significant differences were observed (P = .062) for both groups, with a higher adhesion strength in the air-abraded group compared to the non-air-abraded group, as shown in Table 3.
Regarding the type of failure, the chi-square test showed statistically significant differences (P < .001), with 93.8% of cohesive failures in the air-abraded group compared to 18.8% in the non-air-abraded group. In contrast, the non-surface-treated group had 81.3% adhesive failures compared to none in the treated group. The air-abraded group also had 6.3% mixed fractures while there were none in the non-air-abraded group, as shown in Figure 1.
Discussion
As technology progresses and new polymer materials are developed for fixed prostheses, it is important to understand their bond strength and surface treatment to maximize their service life without debonding. This study aimed to determine the influence of air-abrasion of a 3D printing material for definitive restorations.
The alternative hypothesis can be accepted as air-abrasion provided better bond strength. Surface treatment is important to the adhesion of the material; although there are several surface treatment options, clinically it is common to abrade with different sizes of particles at different pressures. Kang et al in 2023 emphasized that this approach to surface treatment not only serves to eliminate impurities that may remain in the material, but also increases the surface roughness by creating porosity, thereby raising the bond strength; in addition, it causes an increase in the surface energy and wettability of the material, allowing greater penetration of the luting agents into the created surface roughness.15,26 It is for these reasons why the bond strength results obtained in group G1 were higher compared to group G2.
It has been observed in the literature that 50-µm abrasion at 1 to 2 bar pressure creates the surface roughness necessary to have a good adhesion, and a higher surface roughness presents lower SBS results.15,26 This may be related to the fact that the roughness is not uniform and the presence of large and deep porosities left by larger particles or at higher pressure may not be related to good penetration and blocking with the luting agent, which would lead to a reduction in bond strength and long-term bond stability.15 Further studies on 3D printing materials with different particle types are needed to confirm this argument.
Although better results were obtained in the surface-treated group, it should be noted that the results of both groups are in accordance with the bond strength accepted by the ISO 10477:2020 standard for polymeric materials for fixed prostheses,28 which establishes that the bond strength should be greater than or equal to 5 MPa. These results are consistent with those reported in the literature.14,21
In this study, it was decided to thermal-cycle the specimens due to the lack of literature on 3D-printed materials for definitive restorations and the effect of aging.9,12,26 The specimens were subjected to thermal cycling for 5,000 cycles to determine the adhesion strength in the medium term, since this translates to 6 months of clinical use. The methodology for this aging technique is well established in the literature, simulating intraoral changes, without forgetting that it approximates reality, considering only the consumption of hot foods and beverages as well as ice cream.12
This study resulted in slightly lower SBS values than those obtained by Dewan et al,14 although this may be related to the fact that those authors did not thermal-cycle the specimens in their study. Authors such as Kang et al suggest that water acts as a plasticizer on the material, promoting the movement of polymer chains, which causes a change in shape, resulting in decreased mechanical properties.26
Lankes et al argued that the lower adhesion values when the samples are thermal-cycled are caused by the expansion of the polymeric materials due to water, which affects the anchorage of the resin cement, resulting in higher mechanical stress at the bond interface.12 This water effect also interferes with the luting agent. It has been described in the literature that self-adhesive cements tend to absorb water24; this effect of water also interferes with the luting agent, as the literature describes that self-adhesive cements tend to absorb water, which may be another factor in the degradation of bond strength due to volumetric changes in the cement as well as the resin. Nevertheless, further studies with different types of luting agents are warranted.
Studies of bond strength may use different types of tests that simulate different clinical situations. Lankes et al commented that there are studies that question the use of the SBS test,12 but it is a commonly used technique because of its simplicity and low technical sensitivity. However, in the case of materials intended for crown-type restorations, it may be a valid technique, because as Dewan et al noted, this type of SBS force determines the ability of restorations to withstand occlusal forces and the type of material failure.14
Regarding the type of material failure, in the present study, in the G1 group, cohesive failures were mostly observed, as was the case in the study by Lankes et al,12 whereas in the study by Holmer et al,21 more mixed fractures were observed.
An important limitation of this study is that only one surface treatment with a single material and luting agent was compared by thermal-cycling the specimens. Future research should perform the same surface treatment with more types of 3D resin and different resin cements.
Conclusion
Within the limitations of this in vitro study, it can be concluded that: (1) Artificial aging did not seem to influence the shear bond strength between the groups. Nevertheless, there was an improvement in the adhesion on the air-abraded group; and (2) There was a statistical difference between the type of failure, with the cohesive type of failure being the most prevalent in the air-abraded group and the adhesive type in the non-air-abraded group. Uniform failure modes could not be detected in any of the test groups.
ACKNOWLEDGMENT
The authors thank BEGO GmbH & Co. KG for providing the materials used in this study. Their generous contribution was essential to the success of this research.
DISCLOSURE
The authors have no financial interests in the companies mentioned in this study.
ABOUT THE AUTHORS
Jose Gabriel Martinez, DDS, MSc
Clinical Instructor and Trainer, Official Master's Degree in Restorative and Aesthetics Dentistry, Department of Restorative Dentistry, Faculty of Dentistry¸ Universitat Internacional de Catalunya, Barcelona, Spain
Lissethe Peñate, DDS, PhD
Associate Professor, Department of Restorative Dentistry, and Department of Preventive Dentistry, Faculty of Dentistry¸ Universitat Internacional de Catalunya, Barcelona, Spain
María Arregui, DDS, PhD
Assistant Professor, Lecturer in Dental Materials, Department of Dentistry, Faculty of Dentistry¸ Universitat Internacional de Catalunya, Barcelona, Spain
Pablo Atria, DDS, MSc, MPhil, PhD
Clinical Assistant Professor and Director, Advanced Clinical Fellowship in Operative and Digital Dentistry, Department of General Dentistry and Comprehensive Care, New York University College of Dentistry, New York, New York
Julian Conejo, DDS, MSc
Assistant Professor, Clinical Restorative Dentistry, and Director, Chairside CAD/CAM Dentistry, Department of Preventive and Restorative Sciences, University of Pennsylvania School of Dental Medicine, Philadelphia, Pennsylvania