The Use of Nanoceramic Hybrid Material and Digital Light Processing Technology for 3D Printing of an Implant-Supported FP3 Restoration: A Case Report
Marianthi Papaioannou, DDS, MS; Howard P. Fraiman, DMD; Harold S. Baumgarten, DMD; and Michael Bergler, MDT, MS
Abstract: The advancement of digital technologies, including 3-dimensional (3D) imaging and computer-aided design/computer-aided manufacturing (CAD/CAM), and material science has significantly transformed dentistry. Current CAD/CAM techniques incorporate both subtractive and additive (eg, 3D printing) manufacturing methods, with 3D printing offering such benefits as reduced material waste and the ability to produce complex geometries. Much research has focused on the development of hybrid materials that merge the esthetics and durability of ceramics with the high flexural strength, low abrasiveness, and intraoral repairability characteristics of resin composites. Most hybrid ceramics, however, have been optimized for subtractive manufacturing methods, and only a few 3D-printed hybrid materials are available for permanent dental restorations. This case report documents the clinical application of digital technologies in the full-arch rehabilitation of a patient with terminal dentition, highlighting the use of 3D-printed nanoceramic hybrid materials for long-term provisionalization and as a potential permanent solution in the subsequent transition to definitive restorations. The article describes the clinical steps used in the fabrication of the 3D-printed implant-supported restoration, which was clinically tested over 1 year.
The integration of digital technologies such as 3-dimensional (3D) imaging and computer-aided design/computer-aided manufacturing (CAD/CAM) along with advancements in material science has brought transformative changes to restorative dentistry. Subtractive manufacturing technology has long been the standard in CAD/CAM processes; however, 3D printing offers significant advantages, including reduced material waste, greater design flexibility, and the ability to fabricate complex geometries that are challenging or impossible with other manufacturing methods like milling.1,2 The development of novel materials suitable for 3D printing, especially nanoceramic hybrids, is crucial for advancing this technology. Hybrid ceramics aim to combine the esthetic and durability qualities of ceramics with the high flexural strength, low abrasiveness, and intraoral repairability traits of resin composites. Current research, such as a study by Zimmermann et al, highlights the superior fracture resistance of composite materials over ceramics at minimal restoration thicknesses, which suggests that 3D printing could be particularly advantageous in scenarios requiring thin restorations.3
Despite the potential benefits, the mechanical properties of 3D-printed materials often fall short of their milled counterparts. Revilla-León et al found that additively manufactured zirconia exhibits significantly lower flexural strength compared to milled zirconia, raising concerns about the long-term durability of 3D-printed restorations.4 Prause et al similarly noted that 3D-printed composite resins had the lowest biaxial flexural strength among tested materials, with failure often originating from flaws introduced during the printing process.5 Additionally, Çakmak et al reported that subtractive manufactured specimens demonstrated higher microhardness and better color stability than their additively manufactured counterparts.6 These findings challenge the adoption of 3D-printed materials for use in permanent restorations. Thus, while most hybrid ceramics to date have been optimized for subtractive manufacturing methods, only a few 3D-printed hybrid materials are available for definitive dental prostheses.7,8
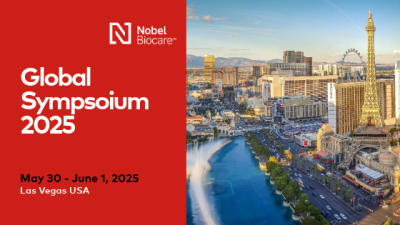
Nobel Biocare Global Symposium 2025
Esthetics also plays a critical role in the success of dental restorations, yet this characteristic remains underexplored in the context of 3D printing. Baumgartner et al successfully used stereolithographic ceramic manufacturing to produce high-density lithium-disilicate glass-ceramics, achieving impressive mechanical properties but with challenges in optimizing esthetic outcomes.9 Further studies by Espinar et al and Çakmak et al revealed that factors such as printing angle, polishing technique, and aging processes significantly influence the color stability and translucency of 3D-printed restorations. These issues highlight the complexity in achieving consistent and desirable esthetic results.10-12
Despite the many significant advancements in prosthetic rehabilitation achieved through 3D printing technology, challenges remain in balancing mechanical integrity and esthetic quality for permanent restorations. As a result, additive manufacturing technology is often limited to trial prostheses due to the scarcity of long-term data on its clinical performance.13 The present case report explores the use of a nanoceramic hybrid material and digital light processing (DLP) technology for the fabrication of an FP3 implant-supported restoration. The restoration was used intraorally for over a year, and during that time its mechanical, esthetic, and biological performance was clinically evaluated. With these findings, the authors aim to contribute to the growing body of information regarding the viability of 3D-printed materials for long-term dental applications and address the ongoing need for reliable, durable, and esthetically satisfying restorative solutions.
Case Report
A 48-year-old woman presented with terminal dentition in both the maxillary and mandibular arches (Figure 1 and Figure 2). The patient's medical history was noncontributory, with no significant systemic conditions or contraindications for dental treatment. Her chief complaints included an inability to chew effectively, which caused significant functional difficulties, and dissatisfaction with her esthetic appearance due to her compromised dental condition.
After a comprehensive clinical and radiographic evaluation (Figure 3), a treatment plan was proposed that included the extraction of all remaining teeth in both arches. Various restorative treatment options were presented to and discussed with the patient. It was decided to proceed with maxillary and mandibular fixed implant-supported restorations.
On the day of extraction, immediate dentures were fabricated and delivered (Figure 4). These dentures provided satisfactory outcomes in terms of function, esthetics, and phonetics, allowing the patient to maintain normal oral functions during the healing phase.
Fully Guided Implant Placement
A 4-month healing period was observed following the extractions to ensure adequate bone remodeling and soft-tissue healing. To facilitate prosthetically driven, fully guided implant placement in the maxillary arch, radiopaque composite resin dots were applied across the surface of the existing maxillary denture (Figure 5). These dentures were scanned intraorally and extraorally using an intraoral scanner to capture both the intaglio surface and external contours.
Additionally, the dentures were mounted (Figure 6) and scanned using a lab scanner to ensure comprehensive data acquisition in terms of the established occlusion and vertical dimension. A cone-beam computed tomography (CBCT) scan was taken with the dentures in place (Figure 7). The standard tessellation language (STL) files from the scans were aligned with the digital imaging and communications in medicine (DICOM) files of the CBCT, creating an accurate digital representation of the patient's oral anatomy.
Based on the integrated digital data, a surgical guide was designed for the flapless, fully guided placement of six maxillary implants. Straight multi-unit abutments of 2 mm height were immediately attached to the implants (Figure 8), followed by the fabrication of an immediately loaded 3D-printed provisional prosthesis (Figure 9).
After a 4-month period to allow for osseointegration, the immediate-load prosthesis was removed. Clinical examination and radiographic evaluation confirmed successful osseointegration of the implants. Conventional open-tray impressions were taken for both the maxillary and mandibular implants after final torque of the multi-unit abutments according to the manufacturer's recommendations. These impressions were used to fabricate 3D-printed verification jigs, incorporating pattern resin for maximum stability to confirm the accuracy of the analog fabricated master models (Figure 10).
Prosthetic Design
The patient's existing temporary restorations, which were satisfactory in terms of function, esthetics, and phonetics, were used as a reference for the design of the final prostheses. The verified master models were cross-mounted with the existing screw-retained temporary prosthesis and scanned using a lab scanner (Figure 11 and Figure 12). A face scan was obtained using a face scanner, allowing for the integration of facial characteristics into the design software (Figure 13). The final prosthesis design was based on the temporary restorations, with minor adjustments made to the shape of the teeth following a discussion with the patient to ensure that the final restorations harmonized with her facial characteristics.
The final design included both the dental structures and the gingival aspect of the restoration in full contour. The prosthesis was printed using a nanoceramic hybrid material (OnX Tough, SprintRay, sprintray.com) and DLP technology. It was printed with a 45-degree angulation on the printing platform to optimize structural integrity and fit (Figure 14). The gingival aspect of the restoration and the dental structures were 3D-stained using a microstaining technique to enhance esthetic outcomes (Figure 15 and Figure 16). Temporary cylinders were cemented according to the manufacturer's protocol. This included sandblasting of the titanium surface with 50-µm aluminum oxide at 1 bar pressure and the application of a metal primer (Alloy Primer, Kuraray, kuraraydental.com). To ensure a chemical bond to the 3D-printed nanoceramic hybrid material, a ceramic primer (Clearfil Ceramic Primer Plus, Kuraray) was applied according to the manufacturer's recommendations. After conditioning both intaglio surfaces, a dual-cure cement (Panavia™ V5, opaque, Kuraray) was used for final cementation of the cylinders into the 3D-printed restoration.
3D-Printed Restoration Insertion
The 3D-printed restorations were inserted, and a thorough fit assessment was performed using radiographic evaluation. Occlusion was carefully evaluated with articulating and shimstock papers to ensure balanced bilateral occlusal contacts in centric occlusion, as well as proper occlusal balance during excursive movements. It was also ensured that the patient could maintain proper oral hygiene with the new restorations. The esthetic results were highly satisfactory and met the patient's expectations (Figure 17).
The patient was scheduled for regular follow-up appointments every 2 months over the course of 1 year. During this period, no gingival inflammation or other complications were observed with the maxillary FP3 prosthesis. However, the mandibular restoration required intraoral repairs after 5 months in the cantilever areas bilaterally due to fracture. The final zirconia restorations were created using the original STL file from the 3D-printed temporary trial prosthesis. This approach ensured that the final restorations were an exact duplicate of the tested temporary prosthesis, including the pink gingival portion of the FP3 prosthesis, without any discrepancies (Figure 18 and Figure 19).
Discussion
The integration of 3D printing technologies into dental practice represents a transformative shift in restorative dentistry, particularly for full-arch implant-supported restorations. Yet, despite the many technological advancements, the application of 3D-printed materials has largely been limited to trial phases, primarily due to a lack of comprehensive long-term data regarding their mechanical durability, biological performance, and esthetic outcomes.13,14
This case report provides insights into the potential of 3D-printed nanoceramic hybrid materials. Over a 1-year evaluation period, the nanoceramic hybrid material demonstrated promising results in terms of both functional performance and esthetic satisfaction. The mechanical limitation that was observed in this case was mainly due to poor performance and lack of resistance to fracture in the presence of cantilevers in the mandibular restoration.
A significant advantage of 3D printing over subtractive techniques is the reduction in material waste. Traditional subtractive methods often result in considerable material loss, whereas 3D printing allows for precise material usage, thereby enhancing the efficiency and cost-effectiveness of the manufacturing process. This reduction highlights the practical benefits of adopting 3D printing technologies in clinical settings.15-18
The esthetic outcome achieved with the 3D-printed nano-ceramic hybrid material was comparable to those produced with conventional materials. The restoration met the patient's expectations, reflecting the advanced capabilities of modern 3D printing technologies to deliver customized, high-quality results. The nanoceramic hybrid material's performance in terms of both durability and esthetics suggests that 3D printing could become a viable alternative to traditional methods, offering enhanced precision and customization.
However, the current limitations of 3D printing materials in clinical practice, including low mechanical strength, surface roughness, and dimensional stability during post-processing, must still be acknowledged and continue to present challenges. Furthermore, the long-term durability of these materials in the oral environment remains a critical concern. The absence of extensive long-term studies means that while the nanoceramic hybrid material showed promising results in this case, further research is necessary to fully understand its long-term mechanical properties and biological behavior.14
Conclusion
The successful rehabilitation demonstrated in this case report highlights the value of a thorough digital approach in contemporary dentistry. The use of 3D-printed nanohybrid ceramics, alongside traditional prosthetic methods, shows potential for addressing long-term restoration needs, including full-arch implant rehabilitations, from both esthetic and biological perspectives. However, the mechanical issue of fracture observed in the mandibular restoration suggests that 3D-printed full-arch implant restorations may face challenges when cantilevers are present. Ongoing advancements in digital technologies and materials are expected to further improve outcomes in implant-supported full-arch rehabilitations. Nonetheless, additional research is needed to assess the long-term mechanical properties and biological factors, such as plaque accumulation, biofilm formation, and monomer leakage, associated with resins.
About the Authors
Marianthi Papaioannou, DDS, MS
Clinical Instructor, Department of Periodontics, University of Pennsylvania School of Dental Medicine, Philadelphia, Pennsylvania
Howard P. Fraiman, DMD
Director, Postdoctoral Periodontal Prosthesis Program, Clinical Associate Professor, Department of Periodontics, University of Pennsylvania School of Dental Medicine, Philadelphia, Pennsylvania
Harold S. Baumgarten, DMD
Clinical Professor, Department of Periodontics, University of Pennsylvania School of Dental Medicine, Philadelphia, Pennsylvania
Michael Bergler, MDT, MS
Adjunct Professor, Division of Restorative Dentistry, University of Pennsylvania School of Dental Medicine, Philadelphia, Pennsylvania