Effect of Implant–Abutment Connection and Abutment Angulations on Peri-implant Stress Levels: A Finite Element Analysis Study
Ishtiyak Khatib, BDS; Nilesh V. Joshi, MDS; Prajakta Rao, MDS; Mridula Joshi, MDS; Anupa Shetty, MDS; Vinayak Thorat, MDS; and Prakash Talreja, MDS
ABSTRACT
Introduction:Placement of an implant in the maxillary anterior region is challenging due to the angulation of bone in this area. Angled abutments may be used to achieve proper restorative contours. The present study was undertaken to examine and compare the stress levels of implants in the maxillary anterior region having different types of internal connections and different abutment angulations. Material and Methods: Implants with three types of abutment connections, internal conical, Morse taper, and internal hex, were modeled using SolidWorks software. Three abutment angulations of 0, 15, and 30 degrees were used for each type of implant. A 100 N axial load was applied to the implants, and the stresses on the implant, abutment, and bone were analyzed by finite element analysis. Results: Among the straight abutments, the most stress was in model 3A (62.60 MPa). The stress value among angled abutments was highest with 30-degree angled abutments. The value was highest in model 3C (94.83 MPa). Internal hex connection showed the highest stress levels in all degrees of angulation of the abutment, and Morse taper connection showed the least amount of stress in all three abutment angles. The most stress concentration was seen in the cortical bone on the buccal surface in the implant-abutment junction. Conclusion: The Morse taper design of implant exhibited the least-highest stress levels on the alveolar bone. The stress levels increased with the increasing angulation of the implant or implant-abutment connection.
When a tooth is extracted or lost from the socket the most substantial changes in the morphology and mass of the surrounding alveolar bone occur within the first 6 months.1 Rehabilitation with a prosthesis within this period helps preserve the quality and quantity of the alveolar bone. Dental implants, because of their property of osseointegration, are considered to be one of the most preferable options biologically for the replacement of missing teeth.2 The prosthesis being fixed requires less-stringent compliance by patients and, thus, is also preferred by them.
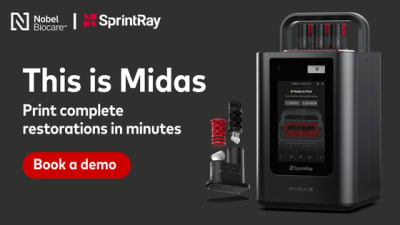
Why Buy Midas from Nobel Biocare
The primary limitations of implants are the need for a surgical procedure and the possibility of failure. Failure of an implant has certain risk factors, such as compromised quality of bone, poor periodontal health, systemic conditions, smoking or parafunctional habits, unresolved dental pathologies, and infections.3 Mechanical factors, including improper load distribution, implant location, inadequate length or number of implants, improper angulations, and lack of osseointegration, also contribute to implant failures.4
The success of a dental implant, therefore, depends on a multitude of mechanical factors involving the load transfer and stresses produced on the implant, abutment, and peri-implant bone. These factors include implant material, geometry, surface structure, design of the implant, and the nature of implant-bone and implant-abutment interfaces. Insufficient vertical and horizontal bone volume are significant challenges for implant placement.5
It is the residual alveolar bone that primarily dictates the long axis and angulations of an implant post-placement. The loss of alveolar bone is amplified in the maxillary anterior region where the amount of horizontal bone resorption has been observed to be double that of vertical resorption.6 Given the spongy nature of maxillary bone, the pattern of alteration in the bone morphology is highly unpredictable, and this can lead to the use of exaggerated angulations in an attempt to address the special needs within the jaw.7 Consequently, implants placed in the thicker cortical plate of the mandibular anterior region were found to have a higher success rate as compared to those placed in the relatively porous spongy maxillary alveolar bone.8
In such scenarios in the maxillary anterior region, when dealing with an undesirable implant orientation that is not parallel to the adjacent teeth, clinicians may use angled abutments to try to achieve proper restorative contours.9 Such intentionally angled abutments have been beneficial in maxillary anterior edentulous spans that comprise severe resorption leading to insufficient bone volume. Another scenario for angulating the abutments is when the implant is placed non-parallel to adjacent teeth/implants either inadvertently or intentionally to avoid contact with important anatomical structures.
A challenge associated with the use of angled abutments is that it leads to increased stresses on the implant-bone level after axial loading.10 Different types of implant-abutment internal connections have been designed to decrease the stresses on peri-implant bone. These include internal hex, internal conical, and Morse taper designs.11,12 Studying these different abutment connection designs can help in determining which of them has beneficial effects on peri-implant bone. Finite element analysis (FEA) is a computer-aided mathematical technique that enables analysis of changes in the shape of any 3D object-in this case bone-based on stress and strain values applied to a material of predetermined properties with a certain value of force.13 FEA has been widely used to analyze stresses on alveolar bone across various branches of dentistry research, including implantology.
In this context, the authors conducted the present study to measure and compare stress levels with abutment angulations of 0 degrees, 15 degrees, and 30 degrees for three different implant-abutment connections-internal hex, internal conical, and Morse taper-in the anterior maxilla using FEA.
Materials and Methods
Creation of a Finite Element Analysis Model
Maxillary bone was modeled to represent the frontal region of the maxilla with 1.5-mm thick cortical bone enclosing a cancellous core, which is similar to D3 bone seen in 65% of the anterior maxillary region.14 Maxillary bone was modeled from a cone-beam computed tomography (CBCT) scan with the aid of SolidWorks 2020 software (SolidWorks, solidworks.com). One representative CBCT of an anterior maxilla having a single missing tooth was used for the study.
Three implants and their corresponding abutments were scanned using reverse engineering technology using the SolidWorks software: Model 1 = internal conical implant-abutment connection; Model 2 = Morse taper implant-abutment connection; Model 3 = internal hex implant-abutment connection. Three different abutment angulations were used for each implant as per the company specifications: approximately 0 degrees, 15 degrees, and 30 degrees (Table 1). Thus, a total of nine models were formed. These were prepared as follows:
Model 1A: Implant with internal conical implant-abutment connection and 0-degree abutment angulation
Model 1B: Implant with internal conical implant-abutment connection and 17-degree abutment angulation
Model 1C: Implant with internal conical implant-abutment connection and 25-degree abutment angulation
Model 2A: Implant with Morse taper implant-abutment connection and 0-degree abutment angulation
Model 2B: Implant with Morse taper implant-abutment connection and 15-degree abutment angulation
Model 2C: Implant with Morse taper implant-abutment connection and 30-degree abutment angulation
Model 3A: Implant with internal hex implant-abutment connection and 0-degree abutment angulation
Model 3B: Implant with internal hex implant-abutment connection and 15-degree abutment angulation
Model 3C: Implant with internal hex implant-abutment connection and 25-degree abutment angulation
Meshing
Meshing is the process of turning irregular shapes into more recognizable volumes called "elements." Meshing is one of the most important steps in performing an accurate simulation using FEA. Mesh generation is the process of dividing the analysis continuum into several discrete parts or finite elements. The finer the mesh, the more accurate the result, although the analysis time is longer (Figure 1).
Applying Loads
100 N of load was applied on the abutment on the cingulum area in the axial direction. When applying a load to the model, in order to stop accelerating infinitely through the computer's virtual ether, a boundary condition was applied. A continuous bond between bone and implant along the entire interface was assumed, and underloading resulted in no relative motion between the bone and implant. This was done to simulate the clinical situation of osseointegration.
The finite element software used in this study was Ansys Workbench (Ansys, ansys.com).
Results
To better visualize the stress state in the structure, the calculated stresses were presented in the form of color bands. Each color band represents a particular range of stress values. The maximum value of stress was denoted in red and the minimum value of stress in blue.
The von Mises stress levels for different types of implant-abutment connections and angulations are collectively summarized in Table 2.
Among the straight abutments, the highest von Mises stress was in model 3A (62.60 MPa). In contrast, the value was lowest with model 2A (32.09 MPa). The stress value rose with increased abutment angulation.
The stress value among angled abutments was highest with 30-degree angled abutments, with the highest value found in model 3C (94.83 MPa) and lowest in model 2C (37.75MPa).
Overall, the stress was highest for 30-degree angled abutments versus any other type of connection and higher on cortical bone as compared to cancellous bone for all models.
The stress levels observed on implant, abutment, implant-abutment junction, and cortical bone by the FEA of the models are depicted in Figure 2 through Figure 4. The results show that stress and strain were mostly concentrated at the coronal one-third of crestal bone, ie, at the implant-abutment joint in the labial surface, while the values declined from the implant-abutment joint toward the apical part of the implant. More stresses were observed to be concentrated in the angled abutment in comparison with the straight abutment. The stress distribution, elastic strain, and deformation in bone were lower in straight abutments as compared to angled abutments. Also, stress and strain were higher on the labial surface compared to the palatal surface of surrounding bone.
Discussion
This study compared the stresses generated by different types of implant-abutment connections and different abutment angulations. Analysis of stress patterns is important in determining where fracture may occur that could subsequently lead to implant failure.15 FEA is a compatible modality for studying the levels and patterns of stress/strain distribution in complex structures such as dental implant-abutment-alveolar bone interfaces.16 The technique has been considered reliable for comparative studies concerning dental implants, with the added advantages of feasibility and accuracy. Hence, the tool was adopted for the present study. The modeling software (SolidWorks 2020) used in this study is a solid modeling computer-aided design (CAD) and computer-aided engineering (CAE) computer program.
Because the alveolar bone density in the maxillary anterior region is generally D3 type, a density similar to this was used in the present study when fabricating the model for alveolar bone.17 Additionally, the available bone height in this region is usually reduced due to anatomical limitations, which contributes to restrictions when determining an adequate length of implant. Therefore, the implant dimensions of 11 mm length and 4.3 mm width, or the nearest possible size according to the implant manufacturer, were modeled in this study. Current evidence recommends the availability of at least 1 mm of bone around the implant for its placement; thus, a peripheral alveolar bone of 1.5 mm buccally and 2 mm palatally was maintained within the models used in this study.
An average of 60 masticatory cycles per minute would account for 2,700 cycles per day. Earlier FEA studies concerning cyclic and static loading on implants have demonstrated that the maximum stress concentration occurs at the abutment neck and cortical bone with the stresses being higher during cyclic loading.18,19 These facts were taken into consideration during calibration of force to 100 N, which was applied to the center of the abutment during FEA.The boundary conditions applied in the model were such that the model bone segment was fixed mesiodistally while the time-dependent dynamic and cyclic (500,000; 1 million; and 3 million cycles) masticatory loads were applied to the cingulum area.9
The results of this study show that higher levels of stress were transferred to the abutments than to the bone. The patterns of stress and strain distribution were greater in cortical bone as compared to cancellous bone. Moreover, stress and strain were mostly concentrated at the coronal one-third of crestal bone, ie, at the implant-abutment joint in the labial surface, while the values declined from the implant-abutment joint toward the apical part of the implant. The strain was higher in the labial surface as compared to the palatal surface of the surrounding bone. The results of the present study are in corroboration with those reported from an earlier FEA of five different implant systems (Ankylos, Astra Tech, Bicon, ITI, and Nobel BioCare) under occlusal loads ranging from 100 N to 300 N.20
In this study the stresses were found to be more concentrated in the angled abutments as compared to the straight type. This could be because with the straight abutments the force generated was along the long axis of the implant and was evenly distributed in and around the implants, whereas in angled abutments the stresses were more concentrated in the bone opposite the abutment angulation, leading to more stress on the buccal cortical bone. These findings were in accordance with earlier reports by Bahuguna et al and Kao et al.21,22 The stress concentration in the cortical bone is opposite to the abutment inclination, which is usually the buccal bone. Therefore, to prevent crestal bone loss, a sufficient thickness of buccal bone should be available when using angulated abutments. Insufficient bone volume may result in buccal fenestration or dehiscence, which can lead to implant failure or unesthetic outcomes.
The amount of von Mises stress around implants increased with the increased angulation of the abutment, which resonated with findings from earlier studies.9,19,23 The higher stress values in angled abutments are due to an increase in stress during off-axis loading. An off-axis load could induce a bending moment and thus exert stress gradients within the implant as well as adjacent bone.24,25 Therefore, off-axis loading must be avoided, and there should be no contacts during eccentric loading of oblique implants. The occlusal contacts need to be limited to centric occlusion as much as possible and all excursive contacts removed to minimize biomechanical effects.19
Morse taper implant-abutment connection was found to produce the least amount of stress among the types compared. This was in line with earlier studies that have reported similar findings.15 The lower stresses generated by this type of implant may be attributed to its geometry, which produces stabilized centralization of stresses along the long axis of the implant. This results in reducing the magnitude of tensions on the cortical bone.
The present study has certain limitations. Clinically, bone is a heterogeneous and viscoelastic material that reacts anisotropically to external forces. In the present study, however, the alveolar bone model used was assumed to be linearly elastic and homogenous, which may not accurately replicate events in a real clinical scenario. Secondly, subjective variations occur during the interpretation of the blending of colors in the FEA model. Furthermore, the masticatory forces in nature are dynamic, whereas the present study was conducted with a uniform axial load of 100 N. Future studies that incorporate in vivo designs and employ dynamic loads may more accurately simulate the orodental mechanism.
Conclusion
Within the limitations of the present study, it can be concluded that the stresses produced on peri-implant alveolar bone increase with the angulation of the abutment. The Morse taper connection leads to the generation of the least amount of stress as compared to the internal hex or conical types of implant-abutment connections. The highest stress values were recorded in the buccal cortical bone opposite the abutment inclination.
ABOUT THE AUTHORS
Ishtiyak Khatib, BDS
Post Graduate Student, Bharati Vidyapeeth (deemed to be) University, Dental College and Hospital, Navi Mumbai, Maharashtra, India
Nilesh V. Joshi, MDS
Professor, Periodontology, Bharati Vidyapeeth (deemed to be) University, Dental College and Hospital, Navi Mumbai, Maharashtra, India
Prajakta Rao, MDS
Professor and Head, Periodontology, Bharati Vidyapeeth (deemed to be) University, Dental College and Hospital, Navi Mumbai, Maharashtra, India
Mridula Joshi, MDS
Professor and Head, Prosthodontics, Bharati Vidyapeeth (deemed to be) University, Dental College and Hospital, Navi Mumbai, Maharashtra, India
Anupa Shetty, MDS
Associate Professor, Periodontology, Bharati Vidyapeeth (deemed to be) University, Dental College and Hospital, Navi Mumbai, Maharashtra, India
Vinayak Thorat, MDS
Associate Professor, Periodontology, Bharati Vidyapeeth (deemed to be) University, Dental College and Hospital, Navi Mumbai, Maharashtra, India
Prakash Talreja, MDS
Associate Professor, Periodontology, Bharati Vidyapeeth (deemed to be) University, Dental College and Hospital, Navi Mumbai, Maharashtra, India
REFERENCES
1. Morjaria KR, Wilson R, Palmer RM. Bone healing after tooth extraction with or without an intervention: a systematic review of randomized controlled trials. Clin Implant Dent Relat Res. 2014;16(1):1-20.
2. Barbier L, Abeloos J, De Clercq C, Jacobs R. Peri-implant bone changes following tooth extraction, immediate placement and loading of implants in the edentulous maxilla. Clin Oral Investig. 2012;16(4):1061-1070.
3. Dawson DR 3rd, Jasper S. Key systemic and environmental risk factors for implant failure. Dent Clin North Am. 2015;59(1):25-39.
4. Do TA, Le HS, Shen YW, et al. Risk factors related to late failure of dental implant-a systematic review of recent studies. Int J Environ Res Public Health. 2020;17(11):3931.
5. Srinath N, Chowdary R, Damodar NA. Challenges and complications in endosseous dental implant: an analysis with case illustrations. J Dent Implants. 2023;13(1):59-67.
6. Tan WL, Wong TL, Wong MC, Lang NP. A systematic review of post-extractional alveolar hard and soft dimensional changes in humans. Clin.Oral Implants Res. 2012;23(suppl 5):1-21.
7. Cavallaro J Jr, Greenstein G. Angled implant abutments: a practical application of available knowledge. J Am Dent Assoc. 2011;142(2):150-158.
8. Ferrigno N, Laureti M, Fanali S, Grippaudo G. A long-term follow-up study of non-submerged ITI implants in the treatment of totally edentulous jaws. Clin Oral Implants Res. 2002;13(3):260-273.
9. Saab XE, Griggs JA, Powers JM, Engelmeier RL. Effect of abutment angulation on the strain on the bone around an implant in the anterior maxilla: a finite element study. J Prosthet Dent. 2007;97(2):85-92.
10. Brosh T, Pilo R, Sudai D. The influence of abutment angulation on strains and stresses along the implant/bone interface: comparison between two experimental techniques. J Prosthet Dent. 1998;79(3):328-334.
11. Grandi T, Cannata M, Samarani RA. A 5-years report from a multicenter randomized controlled trial: dental implants with conical versus internal hex connections. Clin Surg. 2021;6:3322.
12. Bittencourt AB, Neto CL, Penitente PA, et al. Comparison of the Morse cone connection with the internal hexagon and external hexagon connections based on microleakage - review. Prague Medical Rep. 2021;122(3):181-190.
13. Rubo JH, Capello Souza EA. Finite‐element analysis of stress on dental implant prosthesis. Clin Implant Dent Relat Res. 2010;12(2):105-113.
14. Resnik RR, Misch CE. Bone density: a key determinant for treatment planning. In: Resnik RR, ed. Misch's Contemporary Implant Dentistry. 4th ed. Elsevier; 2020.
15. Minatel L, Verri FR, Kudo GAH, et al. Effect of different types of prosthetic platforms on stress-distribution in dental implant-supported prostheses. Mater Sci Eng C Mater Biol Appl. 2017;71:35-42.
16. Eom JW, Lim YJ, Kim MJ, Kwon HB. Three-dimensional finite element analysis of implant-assisted removable partial dentures. J Prosthet Dent. 2017;117(6):735-742.
17. Almasoud NN, Tanneru N, Marei HF. Alveolar bone density and its clinical implication in the placement of dental implants and orthodontic mini-implants. Saudi Med J. 2016;37(6):684-689.
18. Stegarolu R, Khraisat A, Nomura S, Miyakawa O. Influence of superstructure materials on strain around an implant under 2 loading conditions: a technical investigation. Int J Oral Maxillofac Implants. 2004;19(5):735-742.
19. Nokar S, Jalali H, Nozari F, Arshad M. Finite element analysis of stress in bone and abutment-implant interface under static and cyclic loadings. Front Dent. 2020;17(21):1-8.
20. Bozkaya D, Muftu S, Muftu A. Evaluation of load transfer characteristics of five different implants in compact bone at different load levels by finite element analysis. J Prosthet Dent. 2004;92(6):523-530.
21. Bahuguna R, Anand B, Kumar D, et al. Evaluation of stress patterns in bone around dental implant for different abutment angulations under axial and oblique loading: a finite element analysis. Natl J Maxillofac Surg. 2013;4(1):46-51.
22. Kao HC, Gung YW, Chung TF, Hsu ML. The influence of abutment angulation on micromotion level for immediately loaded dental implants: a 3-D finite element analysis. Int J Oral Maxillofac Implants. 2008;23(4):623-630.
23. Martini AP, Barros RM, Júnior ACF, et al. Influence of platform and abutment angulation on peri-implant bone. A three-dimensional finite element stress analysis. J Oral Implantol. 2013;39(6):663-669.
24. Salama AA, Katamesh HA, El Mahallawi O, Halim CH. The effect of platform-switching implants and different abutment materials on the stress distribution of implant-supported restorations. Int J Periodontics Restorative Dent. 2020;40(2):285-291.
25. Desai SR, Singh R, Karthikeyan I, et al. Three-dimensional finite element analysis of effect of prosthetic materials and short implant biomechanics on D4 bone under immediate loading. J Dent Implants. 2012;2(1):2-8.