Use of a Sugar Cross-Linked Collagen Membrane Offers Cell Exclusion and Ossification
Barry P. Levin, DMD; and Yuval Zubery, DMD
Abstract:
Resorbable barrier membranes are advantageous for use in guided bone regeneration for treatment of peri-implant defects, eliminating the need for surgical re-entry. Ribose, a natural sugar-based material that has been used to cross-link collagen, is able to be utilized in high concentrations to extend barrier function for long periods without eliciting inflammation or foreign body reactions in situ. A unique finding with a ribose cross-linked collagen membrane is the in situ ossification of the material upon re-entry. This article presents two cases that clinically demonstrate the efficacy of a sugar cross-linked collagen membrane in peri-implant and site development type defects and suggest its ability to ossify and achieve bone regeneration.
Clinicians have used guided bone regeneration (GBR) as a technique for treatment of peri-implant defects for decades.1 This concept of soft-tissue cell exclusion, facilitating selective cell migration and differentiation, was derived from periodontal research.2,3 The original membrane designed for periodontal and peri-implant defect therapy was nonresorbable and required a second surgical procedure to remove the material.1 This fortuitously provided surgeons an opportunity, however, to evaluate the amount, or lack thereof, of defect resolution/thread coverage. Although this mandatory re-entry was accepted as standard therapy, the disadvantages of it included increased patient morbidity and bone loss associated with full-thickness flap reflection.
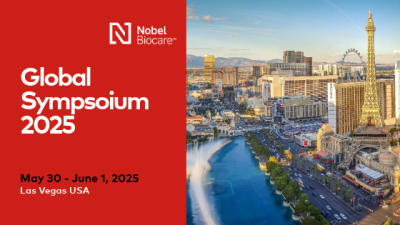
Nobel Biocare Global Symposium 2025
This led to the development of resorbable barrier membranes. The obvious advantages of these biomaterials include the elimination of surgical re-entries to remove the membranes and better bone preservation after regeneration has occurred. Synthetic and natural polymers have been used for these purposes. Of these materials, animal-derived collagen is the most widely used barrier material, as the characteristics that make it favorable to surgeons include: good handling properties, ie, it is pliable, adapts and adheres well to bone, and can be sutured; a relatively high safety profile in that there is a lack of an inflammatory reaction related to breakdown by-products; and rapid collagen breakdown by the host, which decreases the risk of infection and eliminates the need to retrieve the material.4
Regarding barrier function, noncross-linked collagen degrades rapidly and requires replacement in several weeks.5 By cross-linking collagen fibrils, the time it takes for a collagen membrane to break down in situ is extended.6 Through different chemical and physical proprietary processes, attempts are being made to cross-link collagen devices to increase their in vivo life in the tissue. For example, the cross-linking of several barriers is being done using harsh chemicals, such as glutaraldehydes. Because these chemicals are toxic in high concentration, the amount of cross-linking is intentionally limited to avoid adverse tissue reactions that may negatively affect the regenerative outcomes.
Another material used to cross-link collagen is a natural sugar, ribose. The process of glycation closely replicates the manner in which collagen fibrils are cross-linked in the body.7 Being a natural sugar, this material can be used in high concentration that is capable of extending barrier function for long periods of time without eliciting inflammation or foreign body reactions in situ.
Klinger et al demonstrated greater resistance to breakdown with ribose cross-linked collagen membranes after 10 days of exposure to the oral cavity compared to noncross-linked and glutaraldehyde cross-linked collagen membranes.8 In a comparative study, Friedman et al showed a clear advantage to peri-implant defect resolution with a ribose cross-linked membrane compared to a commercially available, noncross-linked barrier.9 This was also demonstrated by Cook and Mealey in an extraction socket model when a bone replacement graft material was covered by a ribose cross-linked membrane without primary closure in humans.10
A unique finding with a ribose cross-linked collagen membrane is the in situ ossification of the material upon re-entry. Not only is significant bone regenerated without inflammatory reactions, but the inner layer of the material appears to serve as a substrate for ossification.11 This has been demonstrated in humans where along with ossification of the membrane, the density of bone in contact with the ribose cross-linked collagen barrier suggested that this material enhanced bone regeneration with a stimulatory effect on neighboring osteoblasts and undifferentiated stem cells.12
This report of two cases demonstrates clinically the efficacy of a sugar cross-linked collagen membrane in peri-implant and site development type defects as well as its ability to ossify and achieve bone regeneration.
Case 1
A 60-year-old woman was referred to the periodontist for treatment of her maxillary right posterior sextant. The existing four-unit fixed partial denture (FPD) required replacement due to a failing mesial (tooth No. 6) abutment (Figure 1 and Figure 2). After sectioning of the bridge between the healthy abutment tooth No. 3 and the pontic in the No. 4 position, a full-thickness flap was reflected. The fractured canine was extracted, preserving the buccal bone at the coronal aspect of the alveolus. Implant placement was performed according to the prosthetic treatment plan. This resulted in a large buccal dehiscence type defect, exposing approximately 8 mm to 9 mm of implant surface (Figure 3).
The defect was obturated with a mineralized bone allograft (OraGraft® FDBA, LifeNet Health, lifenethealth.org) (Figure 4), and a sugar cross-linked collagen membrane (Ossix® Plus, Datum Dental Ltd., ossix-dental.com) (Figure 5) was placed over the defect and beneath the periosteum of the buccal and palatal flaps. The palatal flap was thinned, and a connective tissue pedicle was rotated to cover the exposed membrane over the mesial implant position and sutured to the buccal flap. The buccal and palatal flaps were then approximated and sutured with a monofilament polytetrafluoroethylene (PTFE) suture (Gore-Tex® Suture, Gore, goremedical.com) (Figure 6).
After 10 weeks of healing, the patient returned for surgical uncovery of the two implants and connection of healing abutments (Figure 7). Following a full-thickness flap reflection to confirm osseous regeneration, the remaining collagen membrane was found relatively intact (Figure 8). It was easily removed, revealing complete resolution of the peri-implant defect and a herringbone appearance of the underlying regenerate, resembling the texture of the barrier membrane (Figure 9). The hard, nonpenetrable nature of this specific pattern, along with the white, calcified surface of the tissue, suggested that the membrane may have served as a substrate for ossification, implying the ossification of the inner aspect of the barrier, as demonstrated by Zubery et al.12
After 6 weeks of soft-tissue maturation, the site was restored with a screw-retained, three-unit FPD (Figure 10).
Case 2
A 62-year-old man presented to the periodontist with two symptomatic abutment teeth. These teeth were supporting a provisional bridge, which was deemed hopeless due to the carious and endodontically involved supporting teeth (Figure 11). After flap reflection and extraction of the teeth, the sockets were thoroughly debrided with ultrasonic and manual instrumentation.
Both sites were augmented with the same mineralized bone allograft (OraGraft® FDBA) as used in the first case. Likewise, the same ribose cross-linked collagen membrane as used in the first case (Ossix® Plus) was passively adapted over the surgical site and beneath the periosteum of the buccal and palatal flaps (Figure 12). The flaps were reapproximated without achieving 100% primary closure (Figure 13).
After about 5 months, the site was reopened for implant placement. The superficial layer of the membrane was easily removed, revealing a herringbone-textured, hard bony surface similar to that which was seen in the first case (Figure 14). Because the regenerated bone quality facilitated primary stability, a single-stage healing protocol was selected. After about 10 weeks of healing, restorative therapy commenced. The site was restored with a screw-retained, three-unit, implant-supported FPD (Figure 15).
Discussion
The two cases in this report demonstrate not only the efficacy of a ribose cross-linked collagen membrane, but also its potential to serve as a substrate for ossification. The new bone formed under the grafted space beneath the membrane was of good quality subjectively, allowing for prosthetically guided implant placement, which made it possible for the prostheses to be screw retained. Advantages of this approach include retrievability and avoidance of potential biologic complications related to retained, submucosal cement.
Clearly, regeneration of lost or deficient alveolar bone is critical for successful implant therapy. The advantages of biomaterials that are capable of optimal regeneration without early degradation and soft- or hard-tissue reactions are evident. The early loss of barrier function may compromise regenerative outcomes. This is especially crucial when the membrane is not completely submerged with primary flap closure at the time of augmentation. The second case presented in this article clearly demonstrated the prolonged barrier function of the sugar cross-linked membrane even without primary flap coverage.
This particular barrier is also capable of ossification, as has been demonstrated. Histologic evaluation has shown the affinity for bone formation in proximity to this membrane in sinus augmentations.13 The affinity for osteoblast-like cells for this material has been demonstrated in vitro.14 Compared to noncross-linked collagen, in which the cells are more loosely organized in proximity to the barrier membrane, the sugar cross-linked collagen demonstrated a more dense and organized arrangement of bone-forming cells in vitro.
The ability of the sugar cross-linked collagen membrane to facilitate bone augmentation is an attractive feature to implant surgeons. In a case series, Scheyer and McGuire15 demonstrated similar findings in large peri-implant defects, attributing their success to the extended resorption time and biocompatibility of the membrane.
Conclusion
The current authors found similar outcomes regarding the use of sugar cross-linked collagen membranes to those published by Scheyer and McGuire.15 The ease of handling, resistance to early degradation when exposed, and predictable osseous regenerative outcomes make this material an attractive choice as a barrier membrane in implant practice.
This case presentation demonstrates the possibility of membrane ossification. The cases presented are from a private periodontal practice, where ungrafted controls were not included for ethical reasons. Though this is not a randomized, double-blind, clinical trial, the authors do report consistent findings in their two specialty clinics, with predictable and significant ossification of the membrane and defect resolution.
Disclosure
Dr. Levin has received lecture and consulting compensation from Datum Dental Ltd. Dr. Zubery is Chief Medical Officer for Datum Dental Ltd.
About The Authors
Barry P. Levin, DMD
Clinical Associate Professor, University of Pennsylvania School of Dental Medicine, Philadelphia, Pennsylvania; Private Practice limited to Periodontics and Dental Implants, Jenkintown, Pennsylvania
Yuval Zubery, DMD
Chief Medical Officer and Co-owner, Datum Dental Ltd., Lod, Israel; Private Practice limited to Periodontics and Dental Implants, Ramat Hasharon, Israel