Effect of Finishing Procedures on the Surface Roughness of Resin-modified Glass-Ionomer Materials
Mohammad Garshasb, DDS; Gildo C. Santos, Jr., DDS, MSc, PhD; Amin S. Rizkalla, BSc, MEng, PhD; Richard Bohay, DDS, PhD; and M. Jacinta Santos, DDS, MSc, PhD
Abstract
This study examined the influence of finishing procedures on the surface roughness of different formulations of resin-modified glass ionomers (RMGIs) available in capsules compared with standard resin composites (RCs). Disc samples of three RMGIs and two RCs were fabricated using a metal mold (5 mm x 1.5 mm). Samples were randomly divided into seven groups (N = 10) and subjected to finishing and polishing procedures using a combination of carbide or diamond burs, followed by either rubber points or aluminum-oxide discs. Three different regions of each sample were analyzed using a contact profilometer to determine the average roughness (Ra). The main surface roughness was calculated using a two-way analysis of variance (ANOVA) and the Bonferroni correction for multiple comparisons. A dual-stage combination of a fine carbide bur followed by the use of the finest two grits of aluminum-oxide discs was found to produce the smoothest finished and polished surface. The smoothest surfaces were found to be on the two RCs and one of the RMGIs.
In 1971, Wilson and Kent1 developed a new restorative material based on a glass powder and polyacid solution. The so-called glass-ionomer cement has a glass component, usually fluoroaluminosilicate, which contains a mixture of silica (SiO2), alumina (Al2O3), and calcium fluoride (CaF2).2 The polyacid component of the glass ionomer normally consists of copolymers of acrylic and either itaconic acid or maleic acid.3 These materials are popular mainly because of their ability to bond to dentin and enamel, both mechanically and chemically,3,4 and their coefficient of thermal expansion, which is similar to tooth structure.4 Additionally, these materials release fluoride, providing anticariogenic properties through their incorporation into the tooth structure as fluorhydroxyapatite and having a local anticariogenic effect.5,6 Despite the advantages exhibited by conventional glass ionomers, their disadvantages include poor handling, sensitivity to moisture during initial setting, limited esthetic results, and poor mechanical strength.7 To overcome these drawbacks, resin monomers were added to conventional glass ionomers, resulting in a more resistant material.3,6
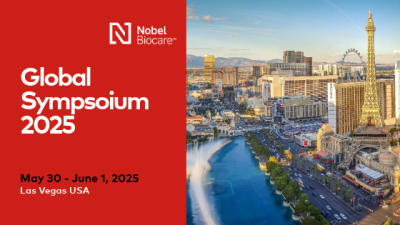
Nobel Biocare Global Symposium 2025
Resin-modified glass ionomers (RMGIs), which are also known as hybrid or dual-cure materials, use a photopolymer that is activated by a light source in addition to the acid-base reaction intrinsic to the glass ionomers’ setting mechanism.8 The addition of resin monomers resulted in increased working time, rapid setting, and greater translucency.8 Furthermore, the incorporation of resin monomers allowed the RMGI restorations to be finished and polished in the same session, eliminating the need for an additional appointment.2 Recently, RMGIs with improved features and easier handling characteristics have been released into the market. Newer versions include RMGIs available in automatic mixing capsules that facilitate handling while maintaining an ideal powder-to-liquid ratio. Additionally, some RMGIs have silane-treated silica nanofillers and nanosized zirconia/silica clusters that appear as a single unit, with a highly packed filler composition.9
The continual improvements made to glass-ionomer materials since their inception have resulted in the development of improved restorative materials with high retention rates.10 The esthetic appearance of these materials, however, is still considered suboptimal.2 To achieve improved esthetic results, an ideal finishing and polishing protocol should be established. The surface roughness of a restorative material is an important factor related to restoration quality and longevity. Smooth, well-contoured restoration surfaces allow for proper esthetics, low gingival irritation, decreased secondary caries, and reduced plaque accumulation.11 Restorative materials have a wide range in surface roughness, which is material-dependent. Therefore, every restorative material needs its own treatment modality to achieve and maintain the desired smooth surface.12
Because of the need to assess the surface characteristics of new versions of RMGI materials, the present study aimed to evaluate the surface roughness of different formulations of RMGI materials submitted to several finishing and polishing procedures. The accepted opinion is that there would be both no difference in surface roughness among the different finishing and polishing tools used and no difference in surface roughness among the different RMGI and RC materials tested.
Materials and Methods
Materials
Three RMGIs available in capsules, GC Fuji II® (GC America Inc., gcamerica.com), Riva Light Cure (SDI Inc., sdi.com.au), and Ketac™ N100 Light Curing Nano-Ionomer Restorative (3M ESPE, 3m.com), and two RCs, Filtek™ Supreme Ultra (3M ESPE) and Aura Universal Restorative System (SDI Inc.) were used in this study (Table 1). A single operator performed standardized finishing and polishing procedures, which were conducted with the instruments listed in Table 2.
Sample Preparation
Disc samples of each material (Table 1) were fabricated using a metal mold with a diameter of 5 mm and a height of 1.5 mm. The materials were carefully manipulated according to the manufacturer’s instructions. After inserting the material, the mold was wedged between two glass slabs (Gold Seal™ Plain Microscope Slides, ThermoFisher Scientific, hermofisher.com) covered with Mylar matrices, and pressure was applied to extrude the excess material to ensure a flat surface. Samples were cured for 40 seconds using a light-emitting diode curing light unit at a light intensity of 1330 mW/cm2 (Bluephase® Style, Ivoclar Vivadent, ivoclarvivadent.com) and stored in distilled water for 24 hours at 37°C. Then, each sample was visually inspected to ensure adequate surface integrity with no physical defects. Samples were randomly divided into seven groups (N = 10), and the finishing and polishing procedures were performed on the top surface of each sample by subjecting the material to one of the following finishing protocols:
G1 – Mylar strip (control group)
G2 – fine diamond bur (Brasseler DET9EF FG Ex - 15 μm, Brasseler USA, brasselerusa.com)
G3 – tungsten carbide bur (Brasseler ET9F FG 16-fluted carbide bur)
G4 – fine diamond bur (Brasseler DET9EF FG Ex - 15 μm), followed by a diamond-impregnated micropolishing rubber point (OptraPol One-Step Polishing System, Ivoclar Vivadent)
G5 – tungsten carbide bur (Brasseler ET9F FG 16), followed by a diamond-impregnated micropolishing rubber point (OptraPol)
G6 – fine diamond bur (Brasseler DET9EF FG Ex - 15 μm), followed by the last two fine grits (ie, light orange and yellow) of aluminum-oxide discs (Sof-Lex™ Extra-Thin Contouring and Polishing System, 3M ESPE)
G7 – tungsten carbide bur (Brasseler ET9F FG 16), followed by the last two fine grits of aluminum-oxide discs (Sof-Lex)
The carbide and fine diamond burs were used in a high-speed handpiece under water spray coolant at a constant air pressure of 40 kg/cm2. Rubber points and aluminum-oxide discs were used in a low-speed handpiece. To standardize the pressure, angulation, and duration of action of the finishing and polishing protocol, a device was developed that allowed for mounting both the sample and the bur handpiece. This device contained a threaded platform that permitted fine adjustments to ensure constant pressure and angulation between the sample and the bur. Each sample was cleaned in an ultrasonic cleaner for 15 seconds between each stage of the finishing procedure. Each of the finishing procedures was applied for 10 passes, and the samples were dried using an air syringe for 10 seconds before surface measurements were made. The fine diamond and carbide burs were replaced after every four samples. The polishing tips and the aluminum-oxide discs were replaced after every sample. Care was taken to standardize every step of the experimental procedures.
Surface Roughness Measurement
Surface roughness was measured using a surface profilometer (Surftest SJ-210 Portable Surface Roughness Tester, Mitutoyo America Corp., mitutoyo.com). The cut-off value for the profilometer was set at 0.25 µm, the stylus speed was set to 0.5 mm/s, and the track length was set to three times. A measuring force of 4 mN with a stylus tip angle of 90 degrees was used. After preparation, each disc was securely affixed onto a glass slab and subjected to assessment by the profilometer. The stylus of the profilometer was placed on the sample, and a path was selected that ensured that the diamond stylus traversed centrally through the sample, excluding the areas located 1 mm from the edge, which were not representative of the finishing and polishing procedures. Three passes were made, each at an angle of 120 degrees to the previous path to ensure a representative sample size.
Profilometer data were analyzed taking the average roughness (Ra) value into consideration. Ra is related to the arithmetical average height of surface component irregularities from the mean line within the measuring length. Scanning electron micrographs (SEMs) were taken to illustrate the surface roughness achieved by the different combinations of materials and polishing methods applied (Figure 1 through Figure 9).
Statistical Analysis
Three different regions were analyzed to determine the Ra, and the main surface roughness for the group was calculated. A two-way analysis of variance (ANOVA) was used to analyze surface roughness with the factors of material and finishing and polishing procedure. Because of significant interaction between the factors, simple main effects were analyzed separately using IBM SPSS Statistics software V.21.0.0 (ibm.com). The Bonferroni correction for multiple comparisons was applied to both factors. The significant P value was set at .01 for material and .007 for method.
Results
Table 3 presents the surface Ra and standard deviations for each material according to the treatment implemented. Figure 10 groups the degree of roughness imparted by each finishing and polishing protocol on each material. Figure 11 shows the surface roughness of each material as a result of the various finishing and polishing protocols used.
Finishing and Polishing Procedures: Single-Stage Protocol
Control—The use of a Mylar strip (control group) generated the smoothest surface for all materials tested. There was no statistical difference in surface roughness between any of the materials used in the control group.
Carbide bur—The use of a carbide bur instead of a fine diamond bur showed decreased surface roughness for the different materials. The roughest surfaces were found to be on the Fuji II (0.74 ± 0.07) and the Riva Light Cure (0.65 ± 0.11) with no significant difference between them. The smoothest surfaces were found to be on the Aura (0.20 ± 0.04), Filtek Supreme Ultra (0.15 ± 0.02), and Ketac N100 (0.17 ± 0.05) with no significant differences between them.
Fine diamond bur—A single-stage fine diamond bur imparted the roughest surface. The material that had the roughest surface after using a fine diamond bur only was the Fuji II (1.07 ± 0.30), whereas the smoothest surface was found on the Filtek Supreme Ultra (0.77 ± 0.48). The Aura had intermediate roughness (0.84 ± 0.41).
Finishing and Polishing Procedures: Dual-Stage Protocol
Fine diamond bur and rubber point—The additional use of a rubber point in conjunction with the fine diamond bur significantly decreased the surface roughness generated compared with the fine diamond bur by itself. The roughest surfaces were found to be on the Fuji II (0.89 ± 0.28), and the smoothest surfaces on the Filtek Supreme Ultra (0.49 ± 0.27) and the Aura (0.50 ± 0.18). Intermediate roughness was found on the Ketac N100 (0.63 ± 0.25).
Fine diamond bur and aluminum-oxide discs—The additional use of the last two fine grits of aluminum-oxide discs in conjunction with a fine diamond bur significantly decreased the surface roughness generated compared with using a fine diamond bur by itself or when using a combination of diamond bur and rubber point. The roughest surface was found to be on the Fuji II (0.68 ± 0.15), and the smoothest surfaces were on the Filtek Supreme Ultra (0.11 ± 0.05) and the Aura (0.14 ± 0.08). Intermediate roughness was observed on the Ketac N100 (0.36 ± 0.19).
Carbide bur and rubber point—The use of a 16-flute carbide bur followed by a rubber point generated the roughest surface on the Fuji II (0.76 ± 0.12). An intermediate roughness was observed on the Riva Light Cure (0.60 ± 0.10). The smoothest surfaces were observed on the Aura (0.13 ± 0.04), the Filtek Supreme Ultra (0.11 ± 0.04), and the Ketac N100 (0.14 ± 0.04) with no significant differences between these groups.
Carbide bur and aluminum-oxide disc—A dual-stage combination of a 16-flute carbide bur followed by the use of the finest two grits of aluminum-oxide discs provided the smoothest finished and polished surface. The smoothest surfaces were observed on the Aura (0.11 ± 0.04), the Filtek Supreme Ultra (0.07 ± 0.02), and the Ketac N100 (0.10 ± 0.03). The roughest surface was found to be on the Fuji II (0.58 ± 0.10). An intermediate roughness was observed on the Riva Light Cure (0.36 ± 0.14).
The dual-stage finishing procedure that used the fluted carbide bur with the aluminum-oxide discs tended to generate the smoothest surface, second only to the use of a Mylar strip. The fine diamond–only protocol generally produced the roughest surface.
Discussion
The most common method for assessing surface roughness involves the use of either a contact or a noncontact profilometer.12 A contact profilometer uses a stylus that moves across the target surface and records vertical displacements across a line or over an area. Average roughness (Ra), which is measured in µm, is then computed and presented as a parameter.12
Because the surface integrity of materials is important to longevity and function, finishing and polishing procedures often are implemented to contour and smooth the restoration. Several tools may be used for these procedures, the most common of which are fluted carbide burs, diamond burs, abrasive stones, rubber points, and aluminum-oxide discs.9 Several finishing and polishing protocols can be implemented for resin-based and RMGI restorative materials. For each of the materials evaluated in the present study, a single-stage finishing procedure using a fine diamond bur consistently generated the roughest surface values. Based on these data, the prevailing opinion that there would be no significant differences between the different finishing and polishing protocols is incorrect. This outcome is in agreement with previous studies that compared diamond and carbide burs.13,14 In the present study, roughness values were at times several orders of magnitude greater than the smoothest surfaces generated. This data is also confirmed pictorially by the SEM micrographs (Figure 1 through Figure 9).
A fine diamond bur–based protocol carved deep trenches into the surface of the restorative material. From a clinical standpoint, the roughness generated by a diamond bur will increase bacterial adherence to a restoration by several fold.15 Bacteria can preferentially colonize rough surfaces, lodge into surface imperfections, and gain protection against shear forces.16 The texture of a surface is said to be smooth when there is minimal vertical deviation between adjacent sections.11 It has been reported that a surface roughness of 0.3 µm can be detected by the tip of the patient’s tongue.17 A previous study reported that there is a threshold surface roughness for bacterial retention (Ra = 0.2 µm) below which no further reduction in bacterial accumulation could be expected.15 Because these roughness values were significantly greater than Ra = 0.2 µm, one would expect a significant simultaneous increase in plaque accumulation and an increase in the risk for both caries and periodontal inflammation, depending on the location of the restoration.18
It has been previously demonstrated that a matrix strip, such as a Mylar strip, creates the smoothest surface on a restoration.19,20 The superiority of the Mylar strip on the surface smoothness was also confirmed in the present study because the Mylar strip protocol consistently generated the smoothest surface for each material. Often the roughness presented after the use of a Mylar strip is appreciably lower than the threshold of Ra = 0.2 µm. Clinically, however, it is usually difficult to achieve ideal shape, contour, and margins without excess by solely using a Mylar strip.19 It is typically necessary to remove excess and reshape the restoration contour after inserting the material in the cavity preparation.
When evaluating the effect of different restorative materials subjected to a single finishing and polishing procedure, the constitution of the material clearly plays an important role in the surface roughness demonstrated. In the present study, materials that used nanofiller particles showed smoother surfaces. To this effect, the Filtek Supreme Ultra consistently demonstrated the smoothest surface for each finishing and polishing category. This result is in agreement with other studies, which reported smoother surfaces for nanofilled materials when compared with microfilled materials subjected to the same finishing and polishing protocols.21-24 On the basis of these findings, the accepted opinion that there are no significant differences in the surface roughness among the different restorative materials tested in the same condition is also incorrect.
Of the RMGIs, the Fuji II consistently presented the roughest surface for each finishing and polishing protocol, followed by the Riva Light Cure restorative material. The Ketac N100 performed similarly to the Aura and Filtek Supreme Ultra RCs for all of the protocols that used a multi-fluted carbide bur. This result is in agreement with a previous study that also observed the smoother surface of a nanofilled glass-ionomer cement when compared with other tested glass-ionomer cements after polishing.25 There was no statistical difference between the surface roughness of the Ketac N100 RMGI and the other RCs for the dual-stage protocols using carbide burs in their first stage. This implies that smoothness comparable with RCs is attainable with RMGIs that incorporate nanofillers.
Although the use of a second-stage finishing protocol has lowered the mean surface roughness values for most materials, for the Ketac N100 RMGI and the Aura and Filtek Supreme Ultra RCs, introducing a second finishing protocol after the use of an initial carbide bur did not yield statistically significant differences. That is to say, a 16-flute carbide bur alone, used on any of these three materials, generated surface roughness values comparable to a dual-stage procedure with mean surface roughness similar to the control protocol using a Mylar strip. These values were equal to or less than the clinically ideal surface roughness threshold of Ra = 0.2 µm.
The use of a single-stage fine diamond bur had a significantly more pronounced effect on the Fuji II and Riva Light Cure restorative materials. When a second-stage finishing and polishing procedure was used after the fine diamond bur, however, the roughness on both of these materials improved, especially when the second stage incorporated the use of aluminum-oxide discs. Other studies have also observed superior finishing of direct esthetic restorative materials with abrasive impregnated discs or aluminum-oxide discs.20,26 Although finishing diamond burs have demonstrated great utility in removing material excess and performing gross contouring, their use should be followed by less abrasive polishing tools, such as rubber points and/or aluminum-oxide discs, which showed great ability in removing the irregularities left by the diamond bur.
Surface roughness can be ascribed to several factors, such as the presence of surface air bubbles, the selective abrasion of the resin matrix, and the exposure of filler particles.9 The coarser particles found in diamond burs tend to pluck filler particles from the surface of a material.27 The localized heat generated during the finishing and polishing procedure can also increase the exfoliation of filler particles, thereby increasing surface roughness, as surface voids are created. Furthermore, these plucked out particles have an additive effect and increase the coarseness of the abrasion.9 Jung28 compared the cutting effectiveness of diamond burs versus carbide burs and found that finishing diamond burs were characterized by high cutting efficiency at the cost of a rougher surface. In contrast, finishing carbide burs generated smoother surfaces but presented lower cutting efficiency.
The use of RCs on anterior and posterior teeth is generally the first option when esthetics and cost are considered. However, RMGIs have gained in popularity because of their important features, such as fluoride release and chemical and mechanical bond to dentin and enamel.3,6 Clinical studies have shown higher retention rates of RMGIs on noncarious cervical lesions when compared with RCs.10 However, RCs are often used because their microsized filler particles have consistently generated the smoothest surfaces. Advances in nanotechnology have opened up the field of material engineering by facilitating a greater degree of control over the chemical and mechanical properties of a substance. These advances have found their way into dental materials, including RMGIs. Nanofillers correspond to primary particles that are 40 nm in size. The decreased size of these particles allows an increased filler load, which has been shown to be associated with better mechanical behavior and higher surface hardness.16,29
When nanoparticles are used the impact of filler plucking and exfoliation is minimized. The plucking of smaller particles creates smaller voids on the surface. Turssi and coworkers22 observed that “nanoclusters were less prone to be sheared off during wear mechanisms,” which has contributed to lesser surface roughness after load. Furthermore, the exfoliation of these smaller particles has less effect on the surface topography than the exfoliation of larger particles.30 Interestingly, Carvalho and colleagues31 observed that nanofilled restorative materials yield intermediate roughness values after chemical and mechanical degradation. This may suggest improved longevity of a restoration incorporating nanoparticles.
Conclusions
Seven different finishing and polishing protocols were used on three RMGIs and two RCs. It was observed that a single-stage finishing and polishing protocol using a fine diamond bur yielded the roughest surface. A dual-stage finishing and polishing procedure using a fluted carbide bur and aluminum-oxide discs yielded the smoothest surface for each material. Among the RMGI materials, the smoothest surface was observed in the one that incorporated nanoparticles (Ketac Nano), and it was comparable to the RCs tested.
About the Authors
Mohammad Garshasb, DDS
Undergraduate Student
Schulich School of Medicine & Dentistry
Western University
London, Ontario, Canada
Gildo C. Santos Jr., DDS, MSc, PhD
Associate Professor
Restorative Dentistry
Schulich School of Medicine & Dentistry
Western University
London, Ontario, Canada
Amin S. Rizkalla, BSc, MEng, PhD
Associate Professor
Chair of the Division of Biomaterials Science
Schulich School of Medicine & Dentistry
Western University
London, Ontario, Canada
Richard Bohay, DDS, PhD
Associate Professor
Oral Medicine and Radiology
Schulich School of Medicine & Dentistry
Western University
London, Ontario, Canada
M. Jacinta Santos, DDS, MSc, PhD
Associate Professor
Schulich School of Medicine & Dentistry
Western University
London, Ontario, Canada