Harnessing the Power of Light Energy
Harnessing the Power of Light Energy
Scott D. Benjamin, DDS
Much has changed since the first laser was approved for use 35 years ago for incising and coagulating soft tissue. Although the early pioneers understood this technology offered great potential for improving treatment outcomes, these innovators probably never imagined the wide range of procedures being implemented in oral healthcare today.
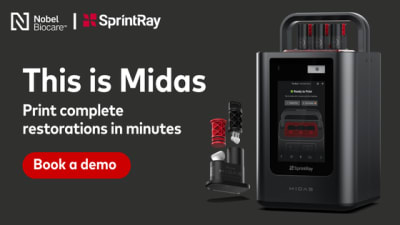
Why Buy Midas from Nobel Biocare
Today, lasers and light-based technologies have become a routine treatment modality for many dental practices. The ever-expanding range of laser procedures include decontamination of periodontal pockets, conservative preparation of teeth for restorations, and advanced procedures such as disinfection of the root canal system, snoring reduction, and management of oral complications from chemotherapy and radiation therapy. Even though the present procedures seem diverse, they are most likely the tip of the iceberg in terms of harnessing the power of light energy to enhance oral healthcare and the related effect to quality of life.
Education
To realize the potential of this technology, a strong foundation is needed. A quality science-based education on the properties of light and composition of the biologic structures is mandatory to enable clinicians and their teams to obtain the best outcomes in patient care. The American National Standards Institute (ANSI) Z136 series on laser safety standards requires device-specific hands-on training for all users of a class 4 laser (a laser capable of removing tissue or damaging the eyes).1,2 In addition, the ANSI standards require that all class 4 laser devices be serviced every 24 months to ensure that the device is operating safely and properly. These requirements apply to all lasers whether they are used in industry, entertainment, commercially, defense, or healthcare.1 In this author’s opinion, some of today’s dental continuing-education programs on lasers are more focused on the device and the selling of the technology than providing the true science of how light interacts with the tissue or laser safety.
Unfortunately, one of the primary impediments to advancing laser technology and incorporating its use into everyday practice is the lack of laser and light-based education in the core curriculum of today’s predoctoral dental programs. As of this writing, Midwestern University’s College of Dental Medicine in Glendale, Arizona, is the only predoctoral dental program that requires their graduates to have both a science-based didactic laser education and clinical competency in laser utilization. The driving force for this program was the dean and administration’s desire to provide the scientific foundation and experience with laser technology. As with all academic education, laser education programs must be focused on science and how to apply that science to the appropriate clinical applications to efficiently enhance outcomes. In addition, Wisconsin is now requiring all dental hygiene schools to incorporate a science-based laser education program and clinical competency in their curriculum. Essentially, more hygiene schools are requiring graduates to have laser competency than dental schools are.
The focus of the American Dental Education Association (ADEA) Special Interest Group on Lasers in Dentistry is to help facilitate the incorporation of the laser education into the academic environment. However, the group recognizes schools have many challenges that they need to overcome to establish a successful program, especially pertaining to laser safety. The 2017 ADEA Annual Session & Exhibition in March at Long Beach, California, has a program focused on how to address and overcome many of the laser safety challenges that schools face and to assist in mitigating these situations.
Laser Science
The law of conservation of energy states that energy is neither created nor destroyed; it is just transferred from one state to another. The basic principles for how laser and light technologies function are relatively simple, as they do only two tasks: They either heat the target or stimulate a response in it. A simple example of this is a curing light. As the light is absorbed in the composite, it stimulates a chemical-reaction curing of the material. The light or photon energy is converted into chemical energy with a side effect of generating heat.
One of the most important features of laser and light-based technologies is the wavelength of the light energy being emitted. Today, a multitude of wavelengths are available for procedures in the oral environment. The array of wavelengths available is from the low 400 nanometers (nm) in the visible light range to invisible thermal rays in the far infrared section of the electromagnetic spectrum with wavelengths higher than 10,600 nm. Unlike the gamma and ultraviolet rays with wavelengths below 400 nm in the ionizing portion of the electromagnetic spectrum that can cause harmful mutagenic changes to the DNA of cells, the wavelengths greater than 400 nm in the visible and thermal portions of the spectrums do not. Combining this with the increased hemostatic properties of most wavelengths with a reduced need for local anesthetics greatly minimizes the contraindications of using a laser and can make it an ideal treatment modality for many patients, especially those who are medically compromised.
The laser’s wavelength determines how efficiently the photonic energy is absorbed in the target tissue (ie, dentin, enamel, bone, soft tissue). The substance that absorbs the light energy is referred to as a chromophore. Obtaining an ideal and efficient outcome requires matching the laser’s wavelength with the chemical composition/chromophore of the biologic tissue that is absorbing the light. This requires the clinician to have an accurate understanding of the chemical composition and structure of the target tissue, which unfortunately is often misunderstood. Water being the primary component of soft tissue comprising over 70% of its volume3 makes it the primary chromophore of that tissue. The more efficiently the wavelength of light is absorbed in water, the more efficiently the tissue is heated. When the water is converted into steam (vaporized), the intracellular pressure increases, the cell explodes, and the cell’s contents are removed as part of the laser plume.
Furthermore, blood is comprised of over 90% water.3 The more engorged the target tissue is with blood, the higher the water content and the more important the wavelength’s absorption in water becomes. The concentration of hemoglobin in blood is only 1.4%4 and even less in tissue. So despite the fact the hemoglobin and melanin highly absorb light in the near-infrared range,5 the concentration of these substances in tissue is so low that they have insignificant roles in heating and vaporization.
The function of lasers in periodontal-pocket decontamination is to inactivate the bacteria and create an environment for the soft tissue to reattach to the cementum of the dentition. To inactivate the bacteria to prevent reproduction, the temperature of the bacteria needs to be elevated to 50° C.6 The chemical composition of bacteria is often misunderstood. Like soft tissue, bacterium is mostly comprised of water, with the percentage of water being as high as 92%.7 The absorption of light in water is an important factor to be considered. It is also commonly suggested that the light energy is absorbed in the pigment of the bacteria. However, black/brown-pigmented bacteria received the name due to their appearance when grown on the agar plate where they obtain the pigment. A study failed to show evidence that bacteria contain any pigment while they are in the periodontal pocket in the oral environment.8
Although selecting the correct wavelength is an important consideration in the treatment process, having the necessary power and the ability to direct it are also important factors. Controlling the interaction of the energy with the tissue varies with the device being used. Studies have shown that wavelengths under 950 nm, which are many of the diodes, are very poorly absorbed in water, the primary chromophore in soft tissue.9 To compensate for this, these devices require that the laser fiber is blackened, often referred to as initiated, with a material such as a cork or articulating paper to absorb the light energy. This is to convert the fiber into a hot-glass tip to heat the tissue with conductive heat transfer. Some lasers in this range state in their operating manuals that the fiber tips should be glowing to be effective.
Diode lasers with wavelengths in 970-nm to 980-nm ranges have approximately a 12-times-higher absorption in water than lasers with wavelengths below 950 nm.9 This enables high-powered 970-nm to 980-nm lasers with peak power levels above 6 watts to use both radiation and conduction heat transfer in a contact mode to interact with soft tissue and enable water convention cooling to assist in the protection of the adjacent tissue.
Similar to the 940-nm lasers, neodymium-doped yttrium aluminum garnet (Nd:YAG) lasers with 1,064 nm have a comparable absorption in water; however, their high peak power approaching as much as 1,000 watts compensates for the reduced absorption in water. The Nd:YAG laser’s extremely short pulse durations enable the use of low average power and control the heat spread and collateral damage into the surrounding tissue.
Carbon dioxide (CO2) lasers with a wavelength of 10,600 nm were the first lasers to be introduced into healthcare in the early 1980s. These lasers have a high absorption in water, substantially more than all the diodes and Nd:YAG lasers.5 CO2 lasers are able to ablate (vaporize) soft tissue efficiently out of contact, using strictly radiant light energy. A key value of these lasers is that they are so efficient that they can either finely focus or defocus the beam of light energy and obtain excellent outcomes for most soft-tissue surgical procedures.
Recently, a CO2 laser has been introduced with a wavelength of 9,250 nm that can be used to perform both soft-tissue and hard-tissue procedures. This is made possible, in part, due to this wavelength’s high absorption both in water and hydroxyapatite.
Hard structures (dentin, enamel, and bone) have a low percentage of water. Lasers that are used to interact with these structures require a high absorption in water. Presently, the lasers that are available for hard-tissue procedures are the previously mentioned CO2 laser with the 9,250-nm wavelength, the erbium chromium-doped yttrium scandium gallium garnet (Er,Cr:YSGG) laser with a wavelength of 2,780 nm, and the erbium-doped yttrium aluminum garnet (Er:YAG) laser with the wavelength of 2,940 nm. The high absorption of these lasers in water causes a micro-explosion with the water rapidly vaporizing, disrupting the crystalline matrix of the hard structures. One of the valuable functions of an Er:YAG laser is a 50-microsecond–pulse width, which is able to disinfect the entire root canal system, enabling the complete obturation of the system, including the accessory canals.10-12
Some interesting and exciting procedures are being performed with lasers and light-based technologies, which are expanding the functions of oral healthcare providers. An Er:YAG laser with specialized handpieces is being used to noninvasively control snoring and enhance the patient’s ability to get a good night’s sleep,13-15 and the Er:YAG laser is being employed to treat the intraoral tissues to tighten facial skin.16
The use of lasers and light technologies for photobiomodulation—the appropriate term for what has commonly been referred to as low-level laser (or light) therapy, or phototherapy—is arguably the one of the exciting aspects of healthcare today. Photobiomodulation is using light energy in a nonablative level to either stimulate or inhibit a response at a cellular level. It is being used to reduce inflammation, control pain, enhance healing, and can aid in the management of temporomandibular joint dysfunction and postsurgical discomfort.
A study being conducted at the University of Pittsburgh Medical Center is showing great promise in controlling and managing the side effect of oral mucositis caused by cancer therapy. A great deal of quality research is being conducted in the area of photobiomodulation, and, in the author’s opinion, the future looks promising.
Selecting the Right Device
As discussed, myriad laser devices are available along with claims of what procedures each device can perform. This has led to conflicting information and confusion for the practitioner who is purchasing a laser. The first question that a clinician needs to ask is: “What are the procedures that I want or need to do immediately?” and “What are the procedures that I would like to perform in the near future and in the long run?” While some lasers can perform many procedures with different efficiencies, there is not a single wavelength or laser that is universal for all procedures. Often, clinicians are using multiple devices to accomplish the desired outcomes for the many procedures that are being performed in today’s dental practices.
A simple key point to always remember is: “The laws of physics do not change.” A common fallacy is that all lasers and all diode lasers are the same. Nothing could be further from the truth, and a good thing to remember is the old phrase: “buyer beware.” When evaluating lasers, several factors need to be considered and addressed. Practitioners need to do their homework to be successful in providing the optimal care for which they strive (Table 1).
About the Author
Scott D. Benjamin, DDS
Past President, Academy of Laser Dentistry
Committee Member, American National Standards Institute Z136 Series of Laser Safety Standards
Private Practice
Sidney, New York