Potential Implication of N-acetylcysteine Detoxification on Adhesive Endodontics
Huang Xueqing, PhD; Zuo Chenqi, MDS; Du Xijin, MDS; Chen Huabing, PhD; Huang Cui, MSc, MDS, PhD; and Franklin Tay, PhD
Abstract
Methacrylate resin-based dentin adhesives and root canal sealers used for bonding of filling materials inside the root canal system are cytotoxic and result in reduction in cell proliferation to a variable extent. An in vitro study to examine the detoxifying effects of N-acetylcysteine (NAC) on four commercial adhesive systems on adhesive-induced cytotoxicity and cell survival was conducted so that the use of methacrylate resin-based materials for filling root canals could be optimized. The finding that NAC co-treatment protected the cells from adhesive-induced toxicity by increasing cellular proliferation, attenuating cell cycle arrest, and reducing cell death suggests the null hypothesis that NAC has no effect on dentin adhesive-induced cell death and cell cycle arrest should be rejected.
Methacrylate resin-based dentin adhesives and root canal sealers are becoming increasingly popular for bonding of filling materials inside the root canal system.1,2 Although methacrylate-based resins perform reasonably well when applied to unexposed vital dentin, they are considerably cytotoxic when applied directly to pulp and periodontal cells.3-5 In vivo studies on direct-pulp capping demonstrate persistent inflammatory reactions, delayed healing, and absence of dentinal bridge formation when dentin adhesives are applied to exposed human pulps.6-9 The adhesives suppress the expression of fibronectin and Type III collagen, which are essential for pulpal repair,10 and they deregulate molecular events involved in the cell cycle, which results in apoptosis and death of pulpal cells.11-13 Because polymerization of dentin adhesives and root canal sealers is difficult to complete, resin monomers and other additives may diffuse from the canal space into the periradicular environment, with the potential of inducing adverse local and/or systemic responses.14,15 When present in high concentrations, these leached resinous components cause depletion of glutathione and stimulate production of reactive oxygen species,15-18 resulting in genotoxicity and cell cycle arrest of the pulpal cells.18-21
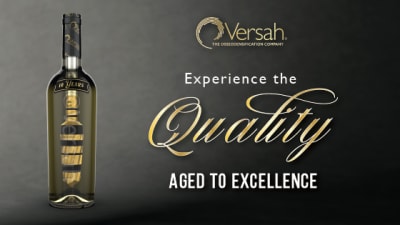
Clinical Versah-tility at your Fingertips
N-acetylcysteine (NAC) is a potent anti-oxidant that is metabolized intracellularly to L-cysteine, a glutathione precursor, and plays a crucial role in intracellular redox balance.22 It protects cultured cells from resin monomer-induced cell cycle arrest and cell death by scavenging reactive oxygen species.23 N-acetylcysteine prevents hydroxyethyl methacrylate (HEMA)-induced apoptosis by inducing cell differentiation, decreasing mitochondrial membrane potential, and inhibiting NF-κB-dependent and independent pathways.19,20,24-26
The compositions of commercial adhesives and root canal sealers are diverse, and various substances may leach after curing.27 Thus, it is pertinent to assess the detoxification effects of NAC on adhesive-induced cytotoxicity and cell survival so that the use of methacrylate resin-based materials for filling root canals may be optimized.28 The present in vitro study examined the detoxifying effects of NAC on four commercial adhesive systems. The null hypothesis tested was that NAC has no effect on dentin adhesive-induced cell death and cell cycle arrest.
Materials and Methods
Extraction of Unpolymerized Resin Components
Two etch-and-rinse adhesives, Prime&Bond® NT™ (DENTSPLY Caulk, www.caulk.com) and Adper™ Single Bond 2 (3M ESPE, www.3MESPE.com), and two self-etch adhesives, G-Bond™ (GC America, Inc., www.gcamerica.com) and Adper™ Easy Bond (3M ESPE), were examined. Polystyrene slides (15 mm x 10 mm) were made from 6-well plate lids and ground with water lubrication using 400-grit silicon carbide paper. After ultrasonic cleaning, the slides were sterilized with ultraviolet light for 30 minutes. Ten microliters of each adhesive were placed separately over the polystyrene slides and light-cured according to the manufacturer’s instruction. After polymerization, the specimens were exposed to ultraviolet light for 1 hour and eluted with 3 mL of Dulbecco’s modified Eagle medium DMEM (HyClone Laboratories, www.hyclone.com) at 37°C for 24 hours in 5% CO2 atmosphere. The sterile extracts were filtered through a 0.22-μm syringe filter (Sartorius AG, www.sartorius.com).
Cytotoxicity Testing
Culture of 3T3 fibroblasts (Hubei-MOST) were conducted using high-glucose DMEM supplemented with 100 units/mL penicillin, 100 mg/mL streptomycin, and 10% fetal bovine serum (HyClone) at 37°C in 5% CO2 atmosphere. The fibroblasts were cultured in 96-well plates at 8x103 cells/well. After 24 hours of incubation, the medium was removed and the cell monolayers were treated with adhesive extracts containing 1 mM, 5 mM, 10 mM, or 15 mM NAC (Sigma-Aldrich, www.sigmaaldrich.com) for 24 hours. Controls consisted of adhesive extracts without NAC. The baseline consisted of cells that were not treated with an adhesive extract. The 3-(4,5-dimethyl-thiazol-2-yl)-2,5-diphenyl-tetrazolium bromide (MTT) assay was used for cytotoxicity evaluation. The culture medium was replaced with 20 µL/well of MTT (0.5 mg/mL; Amresco Inc., www.amresco-inc.com) in phosphate buffered saline (PBS), and the cells were incubated for 4 hours at 37°C in 5% CO2 atmosphere. The MTT solution was replaced with 150 μL/well of dimethyl sulfoxide (DMSO) to dissolve the formazan crystals. The optical density (OD) was immediately measured using a microplate reader at 490 nm. Each treatment was performed in triplicate. Cytotoxicity was expressed as the “relative growth rate,” which was defined as: ([mean OD − mean blank OD]/[mean of baseline OD − mean blank OD]) x 100%.
Cell Viability and Apoptosis
The viability of 3T3 fibroblasts after 24 hours of exposure to the adhesive extracts with or without 5 mM NAC was evaluated via flow cytometry. Floating and adherent cells were harvested by centrifuging and washed twice with PBS. The cells were re-suspended in 200 µL of binding buffer, stained with Annexin V-FITC and propidium iodide (eBioscience, Inc., www.ebioscience.com) and incubated at ambient temperature for 10 minutes. The samples were then diluted in PBS and analyzed using fluorescence-activated cell sorting (FACSCalibur flow cytometer, BD Biosciences, www.bdbiosciences.com). Viable cells (non-staining), apoptotic cells (Annexin V positive) and necrotic cells (both Annexin V and propidium iodide positive or propidium iodide positive alone) were quantified as a percentage of the entire cell population. The sum of apoptotic and necrotic cells was considered as the cell death population.
Cell Cycle Analysis
The G1, S and G2 phases of the cell cycle were determined using FACS analysis of the DNA content. The fibroblasts were harvested by centrifuging after 24 hours of exposure to the adhesive extracts with or without 5 mM NAC. The cells were washed twice with PBS, fixed in ice-cold 70% ethanol, and stored overnight in darkness at -4°C. The cells were then washed twice with PBS, incubated in PBS containing 50 μg/mL RNase at 37°C for 15 minutes, and stained with 50 μg/mL propidium iodide in darkness at -4°C for 1 hour. The samples were then analyzed using the FACS scanner. Assays were performed in triplicate.
Statistical Analysis
Because the normality and equal variance assumptions of the MTT, cell viability and apoptosis, and cell cycle data appeared to be valid, they were analyzed separately using one-way analysis of variance and the Tukey multiple comparison test with the level of significance preset at α= 0.05.
Results
Fibroblasts treated with the adhesives (no NAC, controls) exhibited declines in their relative growth rate in the following order: EO > SB > NT > GB (Figure 1). For all adhesives, the relative growth rate increased in the presence of NAC. The concentration range of NAC for statistically significant reduction in the cytotoxicity of the adhesives appeared to be adhesive-dependent. As 5 mM NAC significantly reduced cytotoxicity for all adhesives, this concentration was employed for the subsequent experiments.
Cell death was observed to varying extents in fibroblasts treated with different adhesives (Figure 2 and Figure 3). The percentage of dead cells induced by NT, SB, GB, and EO were 6.82 ± 0.01%, 8.19 ± 0.46%, 5.61 ± 0.03%, and 28.71 ± 5.87%, respectively. When fibroblasts were co-treated with NAC, cell death was reduced to 6.00 ± 0.16%, 6.88 ± 0.15%, 4.41 ± 0.5%, and 5.09 ± 0.27%, respectively. The percent reduction in cell death in the EO group was statistically significant (P < 0.05). The number of apoptotic cells was less than 4% in all groups.
The cell cycle distribution of 3T3 fibroblasts was adhesive-dependent (Figure 4 and Figure 5). In the absence of NAC, cell cycle was arrested in the G2 phase when the fibroblasts were exposed to NT. By contrast, cell cycle arrest occurred in the S and G2 phases for SB and EO. The percentage of cells in the G2 phase increased slightly in GB but was not significantly different from the non-adhesive-treated baseline (P > 0.05). Inclusion of 5 mM NAC reduced the adverse effect induced by NT on the cell cycle, such that the number of cells in the G1, S, and G2 phases was almost the same as the baseline. For SB and EO, there was a significant increase in the number of cells in the G1 phase and decrease in the number of cells in the S and G2 phases in samples that were co-treated with NAC.
Discussion
The present study indicated that all adhesives were cytotoxic and resulted in reduction in cell proliferation to a variable extent. Cell cycle arrest was also detected in NT, SB, and EO. Conversely, NAC co-treatment protected the cells from adhesive-induced toxicity by increasing cellular proliferation, attenuating cell cycle arrest, and reducing cell death. Thus, the null hypothesis that NAC has no effect on dentin adhesive-induced cell death and cell cycle arrest has to be rejected.
The MTT assay showed that 5 mM NAC significantly improved the relative growth rate in all adhesives (Figure 1). At high concentrations, NAC acts as an antioxidant against oxidative stress, while at low concentrations, it is pro-oxidative and enhances cell damage.23 Schweikl et al found 5 mM and 10 mM NAC were more efficient than 1 mM NAC in protecting cells against HEMA-induced cell cycle arrest.20 Similarly, Stanislawski et al opined that resin-induced glutathione depletion was best alleviated using 5 mM NAC.29 Thus 5 mM NAC was adopted for the subsequent experiments.
The differences in relative growth rates and cell death profiles exhibited by the four adhesives may reflect compositional differences as well as differences in their degree of conversion. The more severe cytotoxicity exhibited by EO and SB may be due to the presence of Bis-GMA30 and HEMA,19,20 which deplete glutathione, causing the cells to generate excess reactive oxygen species.18,19,31 In addition, Bis-GMA induced the expression of hemeoxygenase-1, an oxidative stress responsive gene in pulp cells.30 N-acetylcysteine acts as a free radical scavenger, a precursor to intracellular glutathione and modulates the activity of redox-sensitive cell-signaling and transcription pathways.32,33
A cell cycle may be arrested at different phases to initiate repair of DNA damage or activate the induction of programmed cell death.13 Cell cycle analysis by flow cytometry demonstrated that NT induced cell cycle delay in the G2 phase, while SB and EO induced both S and G2 phase arrest. In these three groups, cell cycle arrest was ameliorated by co-treatment with NAC. Interestingly, NAC has minimal effect on the cell cycle in the HEMA and Bis-GMA-free G-Bond (GB) group (Figure 4). This adhesive was the least cytotoxic among the four adhesives examined (Figure 1). The negligible effect of NAC on disruption of the cell cycle may be attributed to the fewer number of cells exhibiting apoptosis and cell death when fibroblasts were treated with extracts derived from this adhesive in the absence of NAC (Figure 2 and Figure 3).
The finding that Bis-GMA- and HEMA-containing adhesives are considerably more cytotoxic may be extrapolated to methacrylate resin-based root canal primers/sealers in that these components may be present in these materials. The authors do not imply that these materials adversely affect periradicular healing, particularly when they are confined within the root canal space. The results of an in vitro study design based on cell culture should be interpreted with caution, as they are likely to over-exaggerate what may be expected in clinical scenarios. A cell culture environment differs from an in vivo environment in that mechanisms for irritant clearance are lacking and that only one specific cell line is grown to confluency without being segregated by an extracellular matrix. Even when methacrylate resin-based adhesives or sealers are in direct contact with periradicular tissues, suppression of an inflammatory response is unlikely to occur to the extent that results in extensive apoptosis or senescence of the cells derived from the periodontal ligament or the surrounding bone. The results of in vitro cytotoxicity and cell viability studies should only be interpreted as relative indicators of delayed wound healing. Nevertheless, in view of the encouraging results on the reduction in cytotoxicity and cell cycle arrest of HEMA and Bis-GMA-containing adhesives by 5mM NAC, it would be interesting to see if incorporating NAC as a constituent of the final irrigant or as a component in water-containing self-etching primers may result in more favorable wound healing, using in vivo animal models that simulate inadvertent sealer extrusion into the periradicular tissues. Moreover, as adverse pulpal responses and unsuccessful tertiary dentinogenesis have been associated with resin capping of exposed vital pulps, it would be of clinical interest to evaluate if the adjunctive use of NAC in these procedures may alleviate problems that are associated with the cytotoxicity of methacrylate resin-based materials.34,35
NOTE: The first two authors contributed equally to this work.
Acknowledgments
This work was funded by the Nature Science Foundation of China (Grant No. 81070852) and Open Research Fund Program of Hubei-MOST KLOS & KLOBME (200903). The authors thank Michelle Burnside for secretarial support.
About the Authors
Huang Xueqing, PhD
The State Key Laboratory Breeding Base of Basic Science of Stomatology (Hubei-MOST) & Key Laboratory of Oral Biomedicine Ministry of Education, School and Hospital of Stomatology,
Wuhan University
Wuhan, PR China
Department of Prosthodontics
Guanghua School and Hospital of Stomatology and Institute of Stomatological Research
Sun Yat-sen University
Guangzhou, PR China
Zuo Chenqi, MDS
The State Key Laboratory Breeding Base of Basic Science of Stomatology (Hubei-MOST) & Key Laboratory of Oral Biomedicine Ministry of Education
School and Hospital of Stomatology
Wuhan University
Wuhan, PR China
Huizhou Dental Hospital
HuiZhou, PR China
Du Xijin, MDS
The State Key Laboratory Breeding Base of Basic Science of Stomatology (Hubei-MOST) & Key Laboratory of Oral Biomedicine Ministry of Education
School and Hospital of Stomatology, Wuhan University
Wuhan, PR China
Chen Huabing, PhD
College of Life Science and Technology
Huazhong University of Science and Technology
Wuhan, PR China
Huang Cui, MSc, MDS, PhD
The State Key Laboratory Breeding Base of Basic Science of Stomatology (Hubei-MOST) & Key Laboratory of Oral Biomedicine Ministry of Education
School and Hospital of Stomatology
Wuhan University
Wuhan, PR China
Franklin Tay, PhD Department of Endodontics
School of Dentistry
Medical College of Georgia
Augusta, Georgia