All Lasers are Not the Same
All Lasers are Not the Same: Success Requires Knowledge and Training
Just as the practice and procedures of dentistry are not the same as dermatology, plastic surgery, and especially ophthalmology, the use and efficacy of various lasers on the market today also vary greatly. The promotion, popularity, and successful outcomes of laser treatment in these areas of healthcare, particularly in ophthalmology, have made patients and clinicians alike more interested and inquisitive about the use of lasers in dentistry. However, selecting the appropriate device and properly implementing it into practice is not as simple as it seems. The recent introduction of several fairly inexpensive lasers has made this process more perplexing than ever.
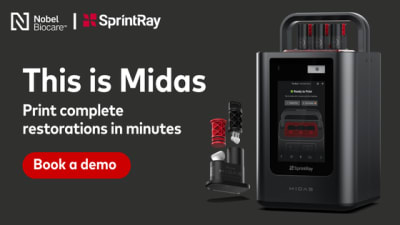
Why Buy Midas from Nobel Biocare
Two of the most frequently asked questions are: “Aren’t all lasers basically the same?” and “Will there ever be one laser that will be the best for doing everything?” The answers to these questions are relatively simple: No, lasers are not all the same, and there will never be one laser that will be the best at everything—unless the laws and principles of physics change. Despite what clinicians hear from sales people, the laws of physics do not change from one product to the next, and the principles of physics are not going to change in the future. Tissue interactions are dependent on the scientific properties of each device. As a healthcare provider, it is your responsibility to learn and understand the scientific principles of every procedure you perform, as well as the laser physics of each device’s use to perform these tasks.
Two Basic Functions
It is the absorption of light energy into the tissue that enables a laser to perform specific tasks and procedures. All lasers perform just two basic functions when they interact with biologic tissue. They either vaporize the target or stimulate a tissue response (such as tissue fluorescence or a healing response). Tissue is vaporized and removed when its temperature is instantaneously raised to its vaporization point and its components become a gas and the cell expands and explodes. This vaporization is accomplished when the laser energy is absorbed into the tissue using either an ablation, incisional, or excisional technique, or, more commonly, with a combination of these techniques.
An incisional technique is performed by applying the light energy in a back-and-forth sawing motion, vaporizing tissue a few cell layers at a time. An excisional technique is used when an amount of tissue is removed in a bulk section such as a biopsy by incising it at its base. An ablation technique is when the light energy is used like a pencil eraser to vaporize (erase) the tissue in a broader area. Almost all surgical procedures are finished by using an ablation technique to sculpture or smooth the tissue.
The wavelength of the light energy emitted by a laser is one of its most important properties and determines how efficiently the light energy is absorbed into the tissue to produce the desired response. The composition of the laser’s active medium is what determines the wavelength of the electromagnetic energy that it produces when the medium is stimulated (Table 1). In recent years, new lasers have been introduced with a semiconductor-active medium that are often referred to as soft-tissue diode lasers. These diode lasers are in the near infrared (NIR) range of the electromagnetic spectrum, with wavelengths ranging from 805 to 1,064 nanometers (nm). Other soft-tissue lasers are carbon dioxide, with a wavelength of 10,600 nm; and Neodymium: Yttrium Aluminum Garnet (Nd:YAG), with a wavelength of 1,064 nm. Hard-tissue lasers, often referred to as all-tissue or Erbium-class lasers, have wavelengths of 2,940 nm for the Erbium: Yttrium Aluminum Garnet (Er:YAG) laser, and 2,780 nm for the Erbium, Chromium: Yttrium, Scandium, Gallium, Garnet (Er,Cr:YSGG) laser.
Matching Wavelength with Chromophore
The components of the tissue that absorb the light energy are called chromophores. One of the primary considerations in the selection of a laser is matching the wavelength of the laser with the chromophores contained in the target tissue (Table 1). The soft tissue of the oral cavity is approximately 70% water, which makes water a primary chromophore for absorption. Hemoglobin and melanin are additional chromophores that play a somewhat minor role due to their substantially lower concentration in soft tissue.
Despite the fact that water is naturally present in the crystals in enamel, dentine, cementum, and bone, the amount is significantly less than the water content of the body’s soft tissue (Table 2). Therefore, a wavelength of light energy is required that is highly absorbed in water, which has peak absorption of light energy at approximately 3,000 nm. In addition, there is a small absorption at around 2,800 nm by carbonated hydroxyapatite mineral of the tissues, but this is far outweighed by the water effects.1 These factors make the Erbium-class lasers with a wavelength of 2,780 nm for the Er,Cr:YSGG and 2,940 nm for the Er:YAG an almost ideal device for the laser vaporization of hard tissues.
When comparing lasers and the light energy they emit, there is a significant difference in their absorption in water. Studies have demonstrated that the energy from a 980-nm wavelength of light energy is absorbed more than 10 times that of an 810-nm light.2 This enhanced absorption of the 970-nm to 980-nm lasers enables the clinician to use water convection cooling to enhance the ablation process and assist in controlling the collateral effects in remaining tissue adjacent to the treatment areas.
Lasers with wavelengths in the 800-nm to 940-nm range compensate for this decreased amount of absorption by initiating the fiber tip (blocking the light energy) with a substance such as articulating paper, ink, or cork, etc, to heat the tip. This hot tip is then used in a contact mode to vaporize the tissue. This method of action prohibits the use of convection cooling during the ablation process, limiting the ability to control the amount of heat dissipation into the remaining tissue.
Taking Control
Besides wavelength, a laser’s interaction with the tissue is also significantly affected by its temporal mode. Controlling the amount of time that the energy is applied will determine the amount of material that it removes and the amount of heat spread into the remaining tissue. All diode and CO2 lasers have the ability to perform in both a pulsed or continuous wave (CW) emission mode. Free-running pulsed lasers (such as Nd:YAG, Er:YAG, and Er,Cr:YSGG) are only able to run in a pulsed mode. In the pulsing mode, the amount of time between pulses is called the thermal relaxation time (TRT). The longer the TRT, the more the remaining tissue is allowed to cool, thus minimizing the thermal effects in the area.
It is extremely important that the clinician has control over the laser duty (emission) cycle, which is the percentage of time that the energy is emitted (on) versus the time off per pulse. The amount of TRT is determined by a combination of the pulse rate expressed in hertz (number of pulses per second) and the duty cycle. Being able to control the TRT gives the clinician an additional modality to assist in attaining the desired outcome for the treatment.
The power that a laser has and how it is controlled is also an extremely important aspect in its efficiency. The peak power of a laser is the maximum amount of energy that is emitted during a single pulse. Some of today’s free-running lasers often have a peak pulse of 1,000 to 2,000 watts. The average power is the amount of energy that is emitted in 1 second and is determined by the peak power and the amount of TRT that occurs during that second. Clinicians are often confused about the different roles and value of peak versus average power. Today’s quality lasers, which are able to manage their high peak power with digital pulsing, give practitioners an even greater ability to use the desired average power to accomplish their goals.
Key Considerations
The science of how a laser performs is only part of what a practitioner should consider when selecting a device. Other considerations include ergonomics, quality of the device and its accessories, cost, and accessibility of consumables (tips, fibers, handpieces, etc). The value of reliability, reputation of the manufacturer, and access to support and maintenance cannot be overstated.
After the science and the investigating of all of the above-mentioned considerations when selecting and evaluating a laser, the single most important and valuable aspect should be device-specific training. Each device is different, with specialized programming and features that need to be thoroughly understood for safe and effective use. Additional education and knowledge from organizations, their websites, and symposiums, such as the Academy of Laser Dentistry (www.LaserDentistry.org) and its annual conference and programs, are also valuable resources that should be utilized for successful implementation.
References
1. Parker SPA, Darbar AA, Featherstone JDB, et al. The Use of Laser Energy for Therapeutic Ablation of Intraoral Hard Tissues. Position Paper: Academy of Laser Dentistry, adopted March 2007. J Laser Dent. 2007;15(2):78-86.
2. Cecchetti W, Guazzieri S, Tasca A, et al. 980-nm diode laser and fiber optic resectoscope in endourological surgery. European Biomedical Optics Week, BiOS Europe, 1996.
About the Authors
Scott D. Benjamin, DDS
Private Practice
Sidney, New York