Regenerative Strategies for Anterior Esthetic Rehabilitation: A Clinical and Histologic Case Report
John Lupovici, DDS
Abstract
In order to solve the substantial challenge of achieving natural esthetics in compromised situations, a number of techniques and materials have been developed to restore deficient alveolar bone and maintain existing bone and soft tissue. This article reviews developments in both areas and reports on a clinical treatment in which a new form of xenograft in conjunction with a cross-linked, cell-occlusive membrane was used in a severely compromised anterior maxilla in preparation for placement of platform-switched implants.
With the rapid advancement in understanding of the biomechanical interaction of implants and bone, implants no longer can be deemed successful simply based on their ability to retain a functional restoration. Instead, the faithful recreation of natural esthetics also has become imperative. Nowhere is that more important than in the anterior maxilla.
Numerous factors may inhibit clinicians from placing anterior maxillary implants in such a way as to yield optimal restorative results. Several clinical and histologic studies have documented the dynamic resorptive process that unfolds after tooth extraction.1-7 The alveolar ridge dimensions may be altered both vertically and horizontally, with up to half the native ridge width lost during the first 12 months and approximately two thirds of that reduction occurring within the first 3 months.3,8,9 This characteristic loss of osseous volume caused by normal remodeling has major consequences for implant reconstructive dentistry, particularly when encountered in the esthetic zone. The ability to obtain an esthetic result is further complicated when the patient presents with compromised native morphology, pathology, trauma, or developmental anomalies.
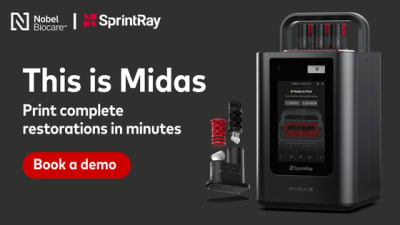
Why Buy Midas from Nobel Biocare
In an attempt to solve some of these challenges, two broad trends have been developed within implant dentistry. The first is the proliferation of techniques and materials available for preventing or regenerating deficiencies in crestal height. The second is the growing understanding of biomechanical strategies for maintaining existing hard and soft tissue, most notably by means of the implant design itself.
Bone augmentation
In horizontally and vertically atrophic ridges, extraction sockets, implant-associated defects, and other anatomic structures that may inhibit proper implant positioning, a number of successful bone regeneration techniques have been well documented. These include ridge splitting and expansion;10-12 guided bone regeneration (GBR);13,14 distraction osteogenesis;15 onlay, inlay, and veneer grafts;16-18 nerve lateralization;19 and sinus augmentation.20,21
When employing such techniques, any bone regeneration material used should optimally be osteoinductive and osteoconductive, easily obtainable, safe, biocompatible, malleable, space-maintaining, and stable after positioning. Several categories of materials have become acceptable alternatives for filling and regenerating bone defects, including autografts, allografts, alloplasts, and xenografts. However, no material meets all the aforementioned criteria.
Autogenous bone, considered historically to be the gold standard, has osseoinductive, osseoconductive, and osteogenic properties and poses no risk of immunologic rejection. Yet, the harvesting of autogenous bone has been associated with various complications and morbidity,22-27 and it may be difficult to obtain in sufficient quantity. High resorption rates and limited viability for autogenous block grafts also have been reported,28-31 and survival rates of implants placed in autogenous grafts have not always exceeded those of implants placed in bone regenerated with alternative materials.32,33
Allograft bone, derived from cadavers and obtained from tissue banks, is the most popular alternative to autogenous bone. It offers the substantial advantage of allowing the patient to avoid a second surgical procedure, with the attendant risks of pain, complications, and morbidity. Available in various shapes and sizes, allograft bone also has fewer supply restrictions than autograft material. However, the donor, tissue bank, and means by which the allograft is processed can dramatically affect its osseoinductive potential (which can range from none to substantial) and the response of the recipient site to its introduction.34-36
Synthetic bone graft materials (alloplasts) constitute a third category of widely used bone-grafting materials. Engineered with properties resembling bone and allowing for bone regeneration, alloplasts include such nonhuman, artificially produced materials as calcium sulfate, calcium phosphate, hydroxyapatite, and bioactive glass. Their advantages consist of their abundant supply, long shelf lives, and lack of potential disease transmission. Excluding a handful of clinical case reports, alloplasts alone have historically displayed evidence of variable clinical successes due to their lack of osteoinductive properties and questionable osseoconductive properties. Their resorption rates may be unacceptably fast or slow, and their capacity to predictably regenerate bone has not yet been demonstrated consistently.
Xenografts constitute the final category of bone-grafting materials in common use. Derived from animal sources, xenografts have been developed largely in response to the donor site complications associated with autografts, as well as the limited supplies of both autogenous and allograft material. Porous bovine-derived material is by far the most common variety of xenograft in use today. Such material has been demonstrated to be highly biocompatible.37 Although concern has also been raised about the possible transmission of bovine spongiform encephalopathy (BSE) by means of xenografts, current data indicate that the risk of BSE transmission is virtually nil.38,39 In fact, no case of BSE traced to long bones from bovine sources has been reported.40
Many of the advantages associated with xenograft material mirror those of allografts. Using bovine bone eliminates the need for a second surgical site and the possibility of any attendant complications. The material is available in various configurations, in unlimited supply, as well as at lower cost than either autogenous or allogeneic bone. It has been demonstrated to be an effective regenerative matrix for periodontal defects, sinus floor elevation, and ridge augmentation prior to implant placement. Histologic analysis has found bovine bone particles to be well incorporated within newly regenerated grafted bone. While some human41 and animal studies42 have concluded that porous bovine bone mineral lacks both osseoinductive and osseoconductive properties, other researchers have reported a high degree of osseoconductivity.37,43-49
For any of the categories of bone-grafting materials, following the principles of GBR and using an appropriate barrier membrane can enhance the likelihood of obtaining optimal results.13 The ideal properties of a barrier for GBR procedures include the ability to exclude unwanted epithelial cells and maintain a space for osteogenic cells to repopulate the graft site. Nonresorbable membranes, while documented to be effective,50 require a second surgical entry and thus increase the patient's cost, discomfort, and psychological stress. Other problems associated with nonresorbable membranes include increased tissue trauma and wound healing complications, such as membrane exposures, infection, bacterial contamination, and poor regenerative outcomes.51-53
Resorbable collagen membranes avoid the drawbacks associated with the need for a second membrane removal surgery and have a number of other benefits. Biocompatible54,55 and nonimmunogenic,55 they promote hemostasis and have been shown to have a chemotactic effect on periodontal ligament cells56,57 and gingival fibroblasts. They promote osteoblast proliferation, as well as increased secretion of transforming growth factor-β1, which is involved in bone remodeling.58 Non-cross-linked resorbable collagen membranes also often foster a very rapid vascularization and excellent tissue reaction subsequent to the vascularization.59,60 However, their resorption profile is roughly only 4 to 8 weeks, which is too rapid, given that a statistically significant increase in bone formation can occur in the 8th to 16th week after grafting (in the absence of complications).61
To extend the absorption profile of collagen membranes, cross-linking techniques have been developed. Cross-linked membranes, while semipermeable and allowing for an exchange of nutrients, have been demonstrated to exclude epithelium and connective tissue for at least 6 months.62 This gives them the potential to provide most of the benefits of nonresorbable membranes, without the latter's drawbacks.
Bone preservation
The most predictable method to achieve a natural appearance prior to implant placement is to preserve the patient's native hard and soft tissue before they are lost. Often, this is not possible; however, the use of minimally traumatic extraction techniques is an important first step in treatment. Another area where vertical bone loss occurs routinely after implant placement is in the peri-implant crestal bone. Bone loss up to the first implant thread has been considered normal since the earliest days of implant dentistry63 and has not threatened the implant's long-term survival. However, as the hard tissue retreats, the soft tissue subsequently is compromised. This consequential loss of soft tissue has a high likelihood of impacting the overall esthetic result.
A number of researchers have theorized the crestal bone loss that typically occurs after connection of a healing abutment is related to development of the biologic width. As bacterial contamination of the implant-abutment junction (IAJ) triggers an inflammatory response, a new biologic width must develop at the IAJ in order to seal off and protect the crestal bone from the oral environment. Thus, the bone recedes to accommodate the peri-implant mucosa.
To forestall this cascade of events, the concept of platform switching has been developed. Platform switching involves the use of a smaller-diameter abutment on a larger-diameter implant collar, a design that shifts the inflammatory cell infiltrate away from the crestal bone. A number of successful long-term clinical cases have been reported, and 1-year postoperative results of clinical research investigations have documented a significant reduction of crestal bone loss around platform-switched implants.64-66
The following presentation illustrates a case in which GBR using a new form of xenograft in conjunction with a cross-linked-collagen cell-occlusive membrane was used to restore a severely compromised anterior maxilla before placement of implants with built-in platform switching in the central incisor positions.
Case Report
A healthy 48-year-old male with a moderate-to-low smile line presented with severe pain above his left maxillary central incisor. A review of his social history revealed that he had smoked approximately one package of cigarettes daily for 15 years. The intraoral examination revealed the presence of a fluctuant mass apical to the maxillary left central incisor and an abutment for an existing fixed three-unit bridge (Figure 1). Radiographic findings confirmed a large (8 mm x 10 mm) periapical radiolucent pathology (Figure 2).
The patient was referred for immediate endodontic evaluation; those findings helped determine that the tooth root had a vertical fracture due to an enlarged post. The right maxillary central incisor was also found to be periodontally compromised. On returning to the surgical office, the patient was advised that smoking has been associated with an increased risk of impaired wound healing and implant failure.67 Nonetheless, he gave informed consent for removal of both maxillary central incisors and immediate reconstruction of the compromised alveolar ridge.
Following administration of local anesthetic (2% lidocaine and epinephrine 1:100,000) and 2 g of prophylactic amoxicillin, impressions were made for on-site fabrication of a provisional restoration. The two central incisors were extracted with minimal trauma to the alveolar bone and both sockets debrided of granulomatous tissue. However, it was not possible to verify conclusively that all the granulation tissue was successfully excised, so a full-thickness mucoperiosteal flap was reflected from canine to canine. This revealed a bony deficiency at the base of the nasal floor measuring approximately 8 mm to 10 mm circumferentially (Figure 3). Due to the minimally traumatic extraction, the thickness of the marginal crestal alveolus appeared to be adequate for primary stabilization of two expanded platform dental implants.
After removal of all remaining granulation tissue, osteotomies were created under copious irrigation within the extraction sites. Two 4/5/4 mm diameter x 11.5 mm NanoTite™ PREVAIL® Implants (BIOMET 3i, https://www.biomet3i.com) were placed, and their stability was verified by torquing both implants to greater than 40 Ncm.
In both sites, the flare of the implant platform effectively obliterated the socket. However, at the left central incisor site, the threads of the implant apex were exposed within the defect (Figure 4). Approximately 0.5 cc of Endobon® Xenograft Granules (BIOMET 3i) were mixed with sterile saline and allowed to hydrate for 10 mins before being introduced into the defect and positioned to fill all void areas (Figure 5). Derived from bovine bone, the Endobon material has been used since 1989 as a bone replacement material in the fields of orthopedic and skull surgery but only recently introduced to the field of oral regeneration. Unlike most xenografts used in oral regenerative procedures, the bone is sintered, a process that involves heating it to more than 1200°C. This removes all pathogenic and organic components from the bovine bone and yields a highly crystalline material containing small amounts of calcium oxide resulting from decomposition of the original carbon content. The sintered bovine bone incorporates macroporosities but preserves the microporosity of the original bone.
A dry 20 mm x 30 mm OsseoGuard® (cross-linked) Resorbable Collagen Membrane (BIOMET 3i) was trimmed to size and placed under the facial flap prior to rehydration. The insertion of the dry membrane allowed the operator to easily verify that the membrane covered the apex of the lesion (Figure 6). It was allowed to hydrate with the patient's blood while under the gingival flap (Figure 7).
Extensive periosteal-releasing incisions were made in the facial flap to allow for passive primary closure. An Essix removable partial denture (DENTSPLY Raintree Essix, https://www.essix.com) was delivered, and it was verified that no contact was being made with the ridge. The patient was given prescriptions for antibiotics and analgesics. He returned for follow-up at 7 days, 14 days, 1 month, 3 months, and 6 months, respectively.
Six months postoperative, a crestal incision with distal release was made and a full-thickness flap was reflected, revealing excellent hard-tissue fill. A 2-mm trephine core was harvested from the facial aspect of the newly regenerated bone and stored in formalin until further histologic evaluation could be performed. Encode® Healing Abutments (BIOMET 3i) were placed into the two internally interfaced implants (Figure 8), and primary closure obtained around the abutments. The Essix retainer was repositioned, and the patient was dismissed.
After 6 weeks of soft-tissue healing, the patient returned for restorative treatment. The definitive three-unit bridge with a cantilever at the right lateral incisor was fabricated and inserted. A radiograph taken at this appointment provided evidence that the crestal height, interproximal bone, and circumferential bone had been preserved, allowing for maintenance of the interproximal papillae (Figure 9 and Figure 10).
Histologic Processing and Examination
The trephine core sample (6 mm in length and 2 mm in diameter) that was harvested at the time of implant uncovering was dehydrated in increasing grades of ethanol and subsequently infiltrated with Technovit® 7200 VLC-resin (Kulzer, https://www.kulzer-technik.de). Following embedding in the acrylic resin, the cylinder was polymerized and sectioned vertically using a cutting-grinding unit (Exakt®, Apparatebau GmbH, https://www.exaktusa.com). The 250-µm thick units obtained were further reduced by microgrinding and polishing to a final thickness of approximately 20 µm to 30 µm. The sections were stained with hematoxylin and eosin and examined under a Leitz DM-RBE® light microscope (Leica, https://www.leica-microsystems.com) equipped with an image system (Q-500 MC®, Leica).
The microscopic examination revealed a number of unresorbed particles, well embedded in the newly formed bone trabeculae. This finding is consistent with histologic examination of other commercially available xenografts (Figure 11 and Figure 12).
Conclusion
The 6-month clinical and histologic results obtained in this case suggest Endobon Xenograft Granules used with OsseoGuard Resorbable Collagen Membranes are viable regenerative materials for cases in which intra-alveolar, space-maintaining deficiencies prevent proper implant placement. Combining these regenerative materials with a platform-switched implant proved to be a successful strategy for anterior esthetic rehabilitation. Further studies with larger patient populations are needed to confirm these results.
Acknowledgments
The author expresses his gratitude to Dr. Joan A. Phelan and Ms. Gloria Turner at the Diagnostic Pathology Laboratory at New York University College of Dentistry.
Disclosure
Dr. Lupovici has received honoraria from Biomet 3i for lectures conducted on the company's behalf.
About the Author
John Lupovici, DDS
Former Clinical Assistant Professor
Department of Periodontics and Implant Dentistry
New York University
New York, New York
Diplomate of the American Board of Periodontology
Private Practice
New York City, New York
Commack, New York