Endosseous Implant: The Journey and the Future
Robert A. Horowitz, DDS; and Paulo G. Coelho, DDS, PhD
In the early 1900s, dentists would insert screw-shaped metallic objects and shells into the alveolar bone to retain or replace teeth. This innovation was further developed by the addition of blades and subperiosteal implants beginning in the 1960s.1 Practitioners used full-arch impressions to have laboratory-fabricated metal frameworks prepared prior to surgical insertion in the bone.2 These were initially made of chrome cobalt and employed to support full-arch prostheses. The procedure description and preliminary clinical report, however, were published with a historical perspective in 1970.3 At that time, patients were restored to function with either segmental or full-arch treatment. Although neither solution had the same success rates as “modern” implants, thousands of patients were helped.
The next generation was comprised of various screw-shaped, biocompatible titanium implants to retain intraoral prostheses. An endosseous implant and the associated surgical technique were designed to firmly anchor the device into the alveolar process and, in ideal circumstances, osseointegrate (bone in close contact with the implant surface).1 The original protocol, in which prior to restoration no functional loading was applied to the endosseous anchor for several months, has resulted in the most reliable treatment modality in dentistry and perhaps medicine.4 While such an approach allowed for high success rates, as patients presented with multiple indications seeking site-specific solutions for anatomic regions with different bone quantity and quality, many design changes in endosseous dental implant systems began.5 Ideally, the device would allow appropriate treatment in any clinical scenario. Implant designing is multidisciplinary, and different aspects are interrelated. The past, present, and what we believe to be the future of endosseous dental implant designs will incorporate macro- and micro-structural design with micro- and nano-technological advances in the field of surface coatings.
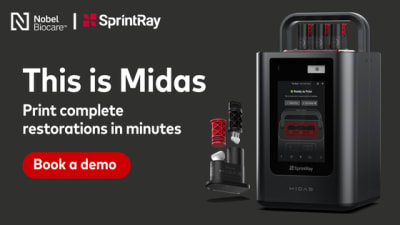
Why Buy Midas from Nobel Biocare
Regarding the endosseous components, two major design features (bulk device geometry and related surgical drilling, as well as surface modifications) have been the most investigated. Because the implant surface is the first part of the device to interact with the host biomolecules, researchers have placed substantial effort into accelerating the host-to-implant response.3,4 Throughout the years, the smoother as-turned surfaces have been replaced by surfaces that, due to either chemical or physical means, are textured—so-called moderately rough surfaces.3,4 A plethora of work has shown that when compared with as-turned surfaces, textured surfaces result in higher degrees of biomechanical fixation, which has encouraged practitioners to decrease the latency period between implant surgery and final restoration. More recently, chemical modification of textured surfaces (ie, processes resulting in increased hydrophilicity or the addition of different ions on the surface or surface oxide) has been proven via in vivo laboratory models to be more effective than their textured predecessors in enhancing the host-to-implant response. Clinical trials are under way to help determine short- and long-term effects on clinical performance.6,7
For more than two decades, highly osseoconductive and bioactive ceramic-coated surfaces have been available as a plasma spray of hydroxyapatite (PSHA). However, the use of PSHA is no longer in favor relative to textured surfaces due to the processing-dependent variation in chemical and physical properties.7 No prospective nor retrospective study has shown lower clinical reliability for such surfaces. To provide benefits from the biocompatible and osseoconductive properties while avoiding potential drawbacks encountered during PSHA manufacturing, researchers have added bioactive ceramics to implant surfaces in the form of nanothickness coatings, discrete deposition, or surface impregnation.8 Relative to textured surfaces, in vivo laboratory studies have shown that bioactive ceramic incorporations have further enhanced the host-to-implant response.7,8 While the dental community is waiting for definitive answers for the clinical effectiveness of newer surface designs, research has been concentrating on two areas. The first ongoing matter concerns relating surface structure to an in vivo property in controlled experiments, which will soon provide data regarding how texture and chemistry should be blended in order to improve host-to-implant response. The second concerns further development of the host-to-implant response by incorporating biomolecules (eg, peptides and growth factors).
The second most investigated design alteration relates to bulk device geometry and related surgical drilling. The stability of the implant at the time of insertion depends on the precise preparation of the osteotomy, bone density, implant shape, and thread design.6 Throughout the years, implant systems have been varied in either the taper of the implant or the shape of and distance between the threads in order to obtain improved fit and early force transfer from the implant to the native bone.6
While healing around dental implant systems presenting diverse surgical protocol is often perceived to follow the same pathway until reaching osseointegration, recent work has shown that healing may substantially differ depending on implant geometry and its relationship with bone immediately following placement.9,10 Recent research has demonstrated that if direct physical contact between implant surface and bone occurs immediately after placement, the bone in contact with the implant surface will undergo remodeling.9,10 Other findings have shown that if voids between the implant bulk and osteotomy wall resulting in a healing chamber exist immediately after implant placement, the chamber will be rapidly filled with woven bone that is shortly replaced by lamellar bone.9,10
Most implant systems are tightly fitted into often-undersized osteotomies, presenting high degrees of primary stability. However, concerns regarding long-term stability have been raised due to interfacial remodeling. The combination of implant geometry and associated surgical drilling, which would provide the highest amounts of stability over time, is desirable. Current trends point toward implant systems that present regions in which direct contact with bone coexist with healing chambers. Narrow-diameter implants take particular advantage of the “wedging” phenomenon, which enhances primary stability. Based on osteotomy size and shape and the aggressive implant taper,11,12 the initial contact of these implants to bone is excellent. The rationale is to obtain high degrees of primary stability during implantation, while attempting to maintain long-term stability as the healing chambers fill with bone during bone remodeling in regions in close contact with bone. Such design approaches are in early development. However, controlling experimental variables, such as thread design, surgical drilling dimensions, and healing chamber size and location, will provide an informed platform design for future implant systems’ macrogeometry and surgical drilling.
So where is the future taking us? Larger biologically active surfaces will help bridge the titanium–bone gap when implants are inserted into clinically challenging weak or regenerated bone. With that, thread patterns and designs will enable implants to be placed in a stable manner that is less traumatic to bone and that will unequivocally maintain and increase such stability over time. We will likely be able to fully reestablish our patients’ anatomies with greater speed and accuracy.
About the Authors
Robert A. Horowitz, DDS
Clinical Professor
Departments of Periodontics, Implant Dentistry and Oral Surgery
NYU College of Dentistry
New York, New York
Paulo G. Coelho, DDS, PhD
Assistant Professor
Department of Biomaterials and Biomimetics
New York University
New York, New York